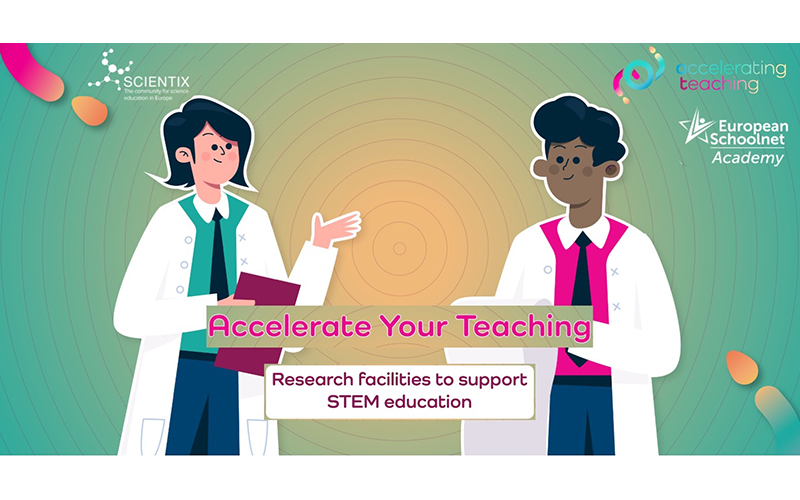
Accelerate your teaching with links to cutting-edge science
Accelerate Your Teaching is a free online course for high-school teachers. Discover how particle accelerator stories can bring a range of STEM topics to life.
The following was adapted from an ILL News article.
Researchers have developed a molecular delivery system for continuous release of an anti-HIV medicine.
Do you remember the last time you took a medicine? It was very likely in the form of a pill. But what actually happens to the medicine in your body after you swallow it?
Once the pill arrives in your stomach, it is broken down to release the medically active molecules that will treat your illness. These molecules travel through the walls of the stomach into your bloodstream. The blood then transports it within your body so that the medicine can reach the organ(s) where it is needed.
At first, the level of the medication in your blood will increase. Over time, the medication will be broken down by your body and eventually excreted, meaning that its levels will decrease again. You can see this increase and decrease in figure 1.
Once the amount in the blood falls below a certain level, the medication is no longer effective. However, high levels need to be avoided because medication can also have harmful side effects. For example, paracetamol, which is used to treat pain and fever, can damage your liver at high doses. Therefore, it is very important that we carefully control the level of medicine in the blood.
In fact, there is a specific dose range corresponding to an amount of medicine which is sufficiently high to treat your disease, but not high enough to harm your body. This range is known as the ‘therapeutic window’ (see figure 2). If we remember that the levels of the medicine rise and fall after you take a pill, as we described above, we see that the blood levels of the medication are only within the therapeutic window for a rather short time.
A very important question is: how can we increase the time span during which the medication level is within the therapeutic window so that the target disease can be treated over a longer time?
There are two options: you can take a higher dose of the medicine or you can take it frequently. This will give rise to medicine blood levels like the ones shown in figure 3. However, a large single dose can increase the medicine blood levels into the toxic range, whereas having to take repeated doses can easily lead to doses being forgotten.
For people infected with the human immunodeficiency virus (HIV), which can cause autoimmune deficiency syndrome (AIDS), this is a particular challenge: to keep the virus under control, it is very important to maintain the medicine level within the therapeutic window at all times. This implies that HIV patients need to take pills every day. Not everybody is able to follow such a strict treatment plan, which can lead to the treatment being inefficient, with serious health consequences.
A very good solution to this problem would be a delivery system that releases an anti-HIV treatment into the bloodstream slowly and continuously (figure 4).
In the past, scientists developed such delivery systems based on peptides (short chains made up of amino acids). The peptides containing the medicine are injected under the skin, where enzymes arrange them into a molecular ‘store’ in the form of a hydrogel, which is a peptide mesh containing water. The medicine is then released slowly from the hydrogel. Unfortunately, peptides are broken down rather quickly by enzymes in the body (figure 5, yellow shapes). To improve these delivery systems, researchers have designed a new type of hydrogel that is made of a mixture of peptides and peptoids (synthetic molecules that resemble peptides but have a different chemical group in the place of the proton on the nitrogen atom in the peptide bond). One of the advantages of peptoids is that they are less well recognized by enzymes that break down peptides, meaning that peptoids are more stable in the body (figure 5, right).
The newly developed peptoid–peptide structures are attached to an anti-HIV medicine (zidovudine, AZT for short) and chemically modified to contain a phosphate group. This group prevents the formation of a gel network until the peptoid–peptide system is injected into the skin, where phosphatase enzymes remove the diphosphate group, which leads to rapid gel formation. The gel network is then hydrolyzed over time, releasing the medicine slowly and continuously into the bloodstream (figure 6).
To optimize their ability to release AZT and get the right dosage, understanding the microscopic structure of the hydrogels is important. First, detailed images of the hydrogels were obtained by using high-resolution microscopy techniques (figure 7). The images show that the hydrogels consist of a three-dimensional sponge-like network of peptoid fibres.
While microscopy shows a snapshot of one specific length scale of the hydrogels, other techniques can provide detailed information over several length scales at the same time. One such technique is small-angle neutron scattering (SANS).
Neutrons are particles that are usually found within atomic nuclei. Free neutrons for experiments (like SANS) need to be produced at specialized spallation sources or at neutron reactors. One such reactor is located at the Institut Laue-Langevin (ILL) in Grenoble, France.
Neutrons produced by the ILL nuclear reactor are a powerful probe to study small samples of materials. They have specific properties that enable them to yield information that is often impossible to obtain using other techniques. Neutrons interact with the nuclei of matter, and observing how they are deflected and change speed gives precise information about the position and motion of the nuclei. Using dedicated instruments, neutrons beams are directed at samples such as the hydrogels described here. The samples scatter some of the neutrons, which are recorded on a special detector. By analyzing how many neutrons are scattered at different angles, researchers can understand the molecular structure and properties of the samples. Importantly, neutrons interact strongly with hydrogen and less so with its isotope deuterium (‘heavy hydrogen’), so if some of the hydrogen in the sample is replaced with deuterium, selected parts of the sample can be highlighted for even more detailed studies.
SANS showed that the molecular structure of the peptoid hydrogels remains the same whether or not they contain AZT. This means that in terms of contributing to the overall structure of the network, the properties of the fibres themselves are more important than the addition of the medicine. It also implies that the network structure is not perturbed by the medicine.
The novel peptoid hydrogels were shown to release AZT over a period of 28 days in test tubes. Further experiments showed that it was absorbed in clinically relevant amounts in an animal model over 35 days. These data mean that the hydrogels are a very good template for the development of long-acting drug delivery systems. Importantly, this technique is expected to be equally efficient in treating a variety of other diseases, such as cancer, malaria, or tuberculosis. Another advantage of these delivery systems is that they can target body parts that are usually hard to access, such as the eyes or the central nervous system.
This work was carried out by researchers from Researchers from Queen’s University, Belfast, with funding from the Wellcome Trust and the UK’s Engineering and Physical Sciences Research Council (EPSRC).
[1] Coutler SM et al. (2024) In Situ Forming, Enzyme-Responsive Peptoid-Peptide Hydrogels: An Advanced Long-Acting Injectable Drug Delivery System. Journal of the American Chemical Society 146: 401–21 416. doi: 10.1021/jacs.4c03751
Accelerate Your Teaching is a free online course for high-school teachers. Discover how particle accelerator stories can bring a range of STEM topics to life.
Camelids are famously robust and useful animals. Surprisingly, their unusual antibodies are just as sturdy and are now revolutionizing medical science.
Play the part: students take on the roles of different components of a synapse to act out synaptic transmission and learn about neurobiology.