Living light: the chemistry of bioluminescence Teach article
Brighten up your chemistry lessons by looking at bioluminescence.
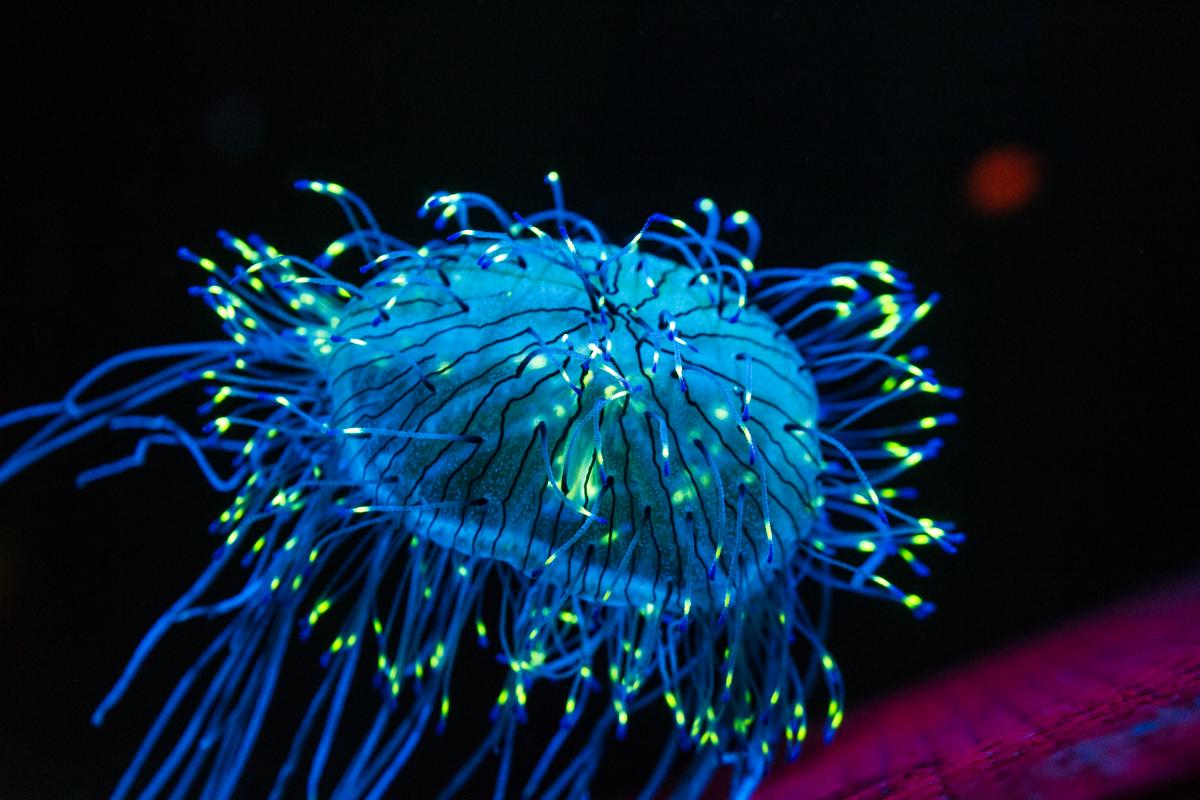
Image courtesy of Chris Favero;
image source: Flickr
Bioluminescent displays are one of the world’s natural wonders. The sheer beauty of the dancing lights from fireflies, or the glowing blue waves caused by ocean plankton, have fascinated people for millennia. While we still find visual delight in such displays today, we are now able to understand the chemistry that makes them happen – and even adapt it for use in the laboratory and elsewhere.
A wide range of organisms, from insects, fish and molluscs to bacteria and plankton, can produce light – as has been known for thousands of years. The Roman author Pliny the Elder described an edible shellfish, Pholas dactylus, that, rather unnervingly, emits light when it is eaten. He also noted that a tree fungus, Omphalotus olearius, produces a brilliant glow at night.
Perhaps the most spectacular bioluminescence displays come from dinoflagellate plankton, which cause the glowing blue waves sometimes seen on the ocean’s surface. More exotic forms of bioluminescence are found in the ocean depths; where there is no sunlight at all, many species make their own illumination. Famously, angler fish use a dangling light to lure their prey straight to their teeth.
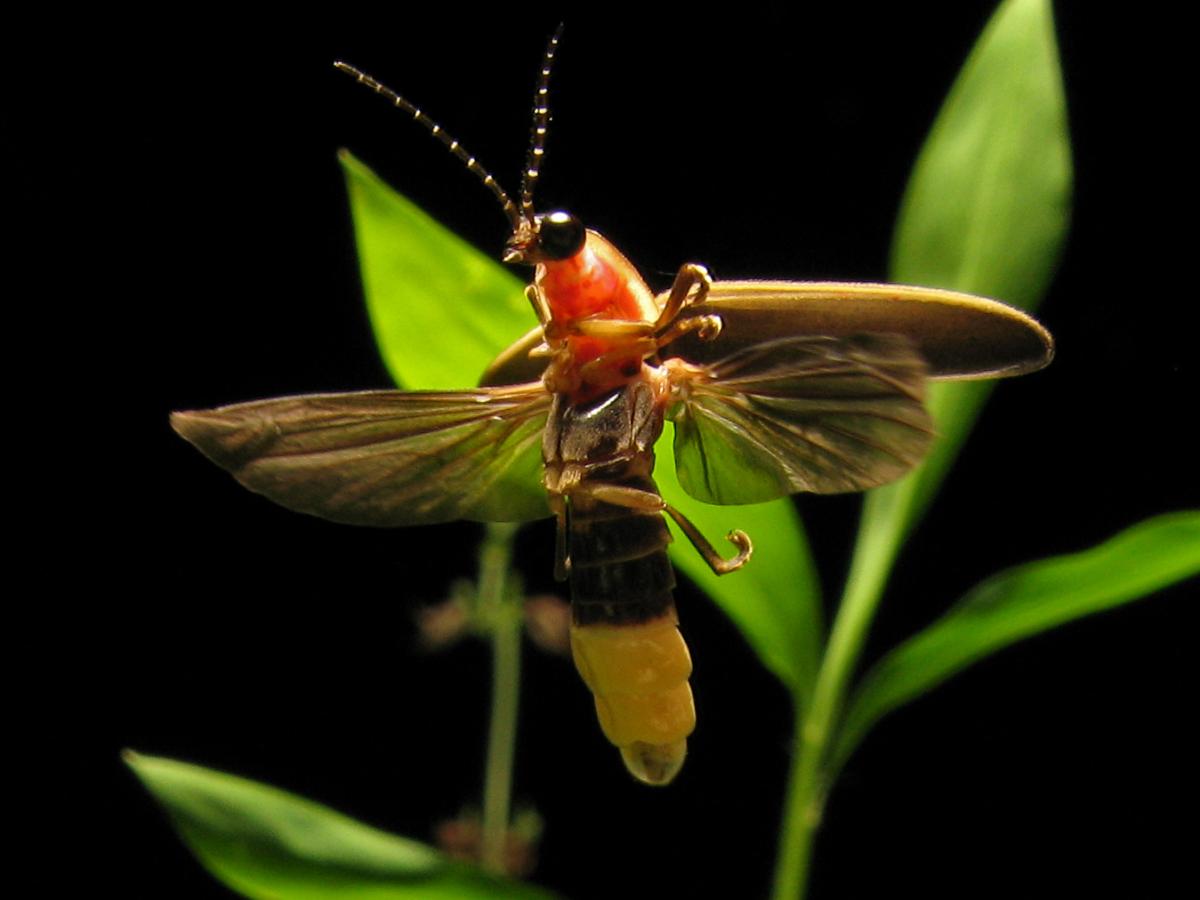
Image courtesy of Terry Priest;
image source: Flickr
Bioluminescence – light produced by living organisms – is widespread in nature, but what advantage does it give the species that use it? In fact, there are many, including:
- Aposematism (toxic appearance) – to look inedible to potential predators. Example: the fireflies Photinus ignitus and Lucidata atra.
- Defence – to startle predators by emitting a bright flash at close range. Example: sternchasers, a type of myctophid or lanternfish.
- Courtship – to communicate before or during mating. Example: fireflies.
- Lures – to attract prey to the light source. Example: the angler fish.
- Camouflage – to help the animal to blend in with its background. As seen from below, a sea animal will look dark against the brightness of the water surface above, so producing its own light will help it to hide from potential predators. Example: squids such as Abralia verany.
Glowing colours
In nature, bioluminescence produces different colours: mainly blue, green and yellow. The distinctive colour of light that a species emits depends on the environment in which it has evolved. Blue emissions usually occur in the deep ocean, green emissions in species that live along the coastline, and yellow (and also green) emissions typically in freshwater and terrestrial species.
What is the chemistry that makes bioluminescence happen? And how are the different colours – blue, green, yellow – obtained?
Chemically, most bioluminescence is due to oxygenation reactions: oxygen reacts with substances called luciferins, producing energy in the form of light. The reactions are catalysed by enzymes known as luciferases. In this process, the luciferins become oxygenated to form oxyluciferins. As table 1 shows, the luciferins used by different species and the resulting oxyluciferins can be quite varied chemically.
Bioluminescent species | Luciferin | Oxyluciferin |
---|---|---|
Dinoflagellates | Dinoflagellate luciferin
C33H3806N4Na2 ![]() |
![]() |
Squid, some shrimps, some fish | Coelenterazine
C26H2103N3 ![]() |
![]() |
Fireflies | Firefly luciferin
C11H8N2O3S2 ![]() |
![]() |
These reactions are very efficient, with about 98% of the energy involved being released as light. This compares with an efficiency as low as 2% for a traditional filament light bulb, which also releases large amounts of energy as heat.
As well as occurring in different species, some luciferins can produce more than one colour of light (see table 2). Additional light-emitting substances, or fluorophores, can also change the colour of the luminescence. The jellyfish Aequorea victoria contains one such fluorophore, known as green fluorescent protein (GFP). GFP absorbs the blue light produced by the initial reaction and re-emits it at a longer wavelength as green light, so the jellyfish produces a green bioluminescence.
Luciferin | Luminescence maximum (nm) |
Approximate colourw1 |
|
---|---|---|---|
Firefly luciferin | 560 (at pH=7.1) | Green |
![]() |
615 (at pH=5.4) | Orange |
![]() |
|
Bacterial luciferin | 490 | Turquoise |
![]() |
Dinoflagellate luciferin | 474 | Blue |
![]() |
Coelenterazine | 450-480 as an anion | Blue to turquoise |
![]() |
400 in the –COOH form | Purple |
![]() |
In recent decades, this particular bioluminescence system has found an important use in scientific research: the gene that encodes GFP is now used as a genetic ‘tag’ to trace specific proteins and to reveal when particular genes are expressed. Because GFP glows green under blue or UV light, it is very easy to detect (see Furtado, 2009). This work was considered so important that it was awarded the Nobel Prize for Chemistry in 2008w2.
Fortunately for us, it is quite easy to replicate in the laboratory the type of chemical reaction that causes bioluminescence, as the activity below demonstrates.
Student activity: bioluminescence in the laboratory
In this activity, students can see a luminescence reaction take place when chemical reagents are mixed together. The key ingredient is luminol, a synthetic chemiluminescent substance that produces a blue glow when it reacts chemically. Although the reactions of luminol and luciferin are different – the oxidation reaction of luminol is catalysed by potassium ferricyanide rather than by an enzyme (for more details, see Welsh, 2011) – the result is the same: luminescence.
The final step in the activity should be carried out in a dark place to make the most of the light show.
Materials
- 1 g luminol (5-amino-2,3-dihydrophthalazine-1,4-dione)
- 50 ml sodium hydroxide (NaOH) 10% w/w solution
- 50 ml potassium ferricyanide (K3[Fe(CN)6]) 3% w/w solution
- Approximately 0.5 g potassium ferricyanide (K3[Fe(CN)6])
- 3 ml hydrogen peroxide (H2O2) 30% m/m solution
- Distilled water
- Beakers
- Funnel
- Cylinders
- Flask
Procedure
Safety note
Safety glasses, a lab coat and safety gloves should be worn. Care should be taken when handling the 30% hydrogen peroxide solution, as this can react violently in the presence of a catalyst. Close the bottle as soon as you have removed your 3 ml of solution.
See also the general Science in School safety note.
- In a beaker, dissolve 1 g luminol in 450 ml distilled water.
- Add 50 ml 10% sodium hydroxide solution and mix.
- Take 50 ml of the resulting solution and add it to 350 ml distilled water in another beaker. This is now Solution A.
- In a third beaker, mix 50 ml 3% potassium ferricyanide solution with 350 ml distilled water and 3 ml 30% hydrogen peroxide solution. This is Solution B.
- Pour equal amounts of Solutions A and B into separate cylinders.
- Put some potassium ferricyanide into the flask, and place the funnel on the flask.
- Move the flask to a dark place.
- Pour Solutions A and B into the flask at the same time, and watch what happens.
A wonderful light-blue luminescence will start at once!
- Disposal: heat the solution in a fume cupboard to concentrate it until the volume is 1/8 of the initial amount, then pour the remaining solution into the heavy metal residuals tank.
What is happening?
The oxidation of luminol occurs in several steps.
- While Solution A is being prepared (step 2), luminol reacts with the base (OH–):
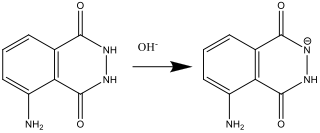
- When Solution B is prepared (step 4), hydrogen peroxide decomposes to form the superoxide radical anion O2.-. This reaction is catalysed by the hexacyanoferrate (III) ion.
- When Solutions A and B are mixed (step 8), luminol is oxidised by the hexacyanoferrate (III) anion, forming a radical anion:
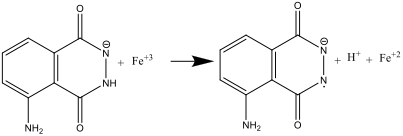
The hexacyanoferrate ion therefore has a dual role: it catalyses the formation of the superoxide radical anion, O2.- , and also oxidises luminol into a radical anion. The iron needs to be in the form of a complex such as [Fe(CN)6]-3, to prevent the precipitation of Fe(OH)3 in the strongly alkaline environment.
- The luminol radical anion and the superoxide radical anion, O2.-, then react:
- The resulting compound is unstable and decomposes to produce nitrogen and an excited form of the aminophtalate ion:
- The excited form then transforms and decays into a stable form, emitting the energy difference as light:
Questions for discussion
- In the luminol reaction, where does the energy for the light come from?
- What is the role of the potassium ferricyanide? In natural luminescence reactions, what substance performs this role?
- Which substance is responsible for oxidation in the luminol reaction? Is this the same in nature?
Extension
There is a great deal of information available about bioluminescence (see the resources section for examples). Students can follow up this activity with some research of their own. For example:
- Uses of bioluminescence in nature. Find more reasons why bioluminescence is a useful adaptation. For each adaptation, identify some species that benefit from it.
- Bioluminescence chemistry. Find out about some specific chemiluminescence reactions that occur in nature. How similar are they to the luminol reaction?
- Bioluminescence colours. Find out more about how they are produced.
- Evolution of bioluminescence. Has it evolved many times or just once?
- Uses of luminol in crime-scene investigations. Find out how luminol is used forensically and the chemistry of this use (e.g. see Welsh, 2011).
Studying chemistry with Pliny the Elder
This activity is part of a larger interdisciplinary project, developed together with 14- to 15-year-old students, to explore ancient scientific techniques. Pliny the Elder (23-79 AD) was a Roman author and naturalist whose encyclopaedia, Naturalis Historia, brought together much of the scientific knowledge of the time. We began each topic by discussing a passage from Naturalis Historia and then worked out how to recreate either the experiment described in the text or something similar.
In this way, the students began in the same pre-scientific state as Pliny and, through laboratory work and discussion, gained modern scientific knowledge on each of the topics. The process motivated even the most unenthusiastic students.
Other activities in the project include synthesising indigo (Farusi, 2012), recreating ancient perfumes (Farusi, 2011), preparing glass tesserae with boric acid and preparing iron-gall ink (Farusi, 2007). The whole project was presented at the international Science on Stage festivalw3 in Copenhagen, Denmark, in 2011.
References
- Farusi G (2007) Monastic ink: linking chemistry and history. Science in School 6: 36-40
- Farusi G (2011) Smell like Julius Caesar: recreating ancient perfumes in the laboratory. Science in School 21: 40-46
- Farusi G (2012) Indigo: recreating Pharaoh’s dye. Science in School 24: 40-46
- Furtado S (2009) Painting life green: GFP. Science in School 12: 19-23
- Welsh E (2011) What is chemiluminescence? Science in School 19: 62-68
Web References
- w1 – A simple tool to convert a wavelength in nanometres to an RGB or hexadecimal colour is available on the Academo website, a free collection of educational resources
- w2 – An accessible account of the 2008 Nobel Prize for Chemistry awarded to Osamu Shimomura, Martin Chalfie and Roger Y Tsien is available on the Nobel Prize website
- w3 – Science on Stage is a network of local, national and international events for teachers, initially launched in 1999 by EIROforum, the publisher of Science in School. At each national event, a delegation of teachers is selected to represent their country at the Science on Stage international teaching festival. During the festival, about 350 primary- and secondary-school teachers from 25 countries will share their most innovative teaching ideas in workshops, on-stage performances and the teaching fair.
The next international festival will be held on 29 June – 2 July 2017, in Debrecen, Hungary. Participants will be chosen through competitive national events in 25 countries. To find out more information about the application process, contact your national steering committee.
Resources
- Two accessible articles about (bio)luminescence:
- Douglas P, Garley M (2010) Chemistry and light. Science in School 14: 63-68
- Judson O (2015) Luminous life. National Geographic
- A charming and informative animation about bioluminescence
- An atmospheric video with music celebrating the extraordinary beauty of ocean bioluminescence
- A short video demonstrating how to make luminol
- A longer video showing how to make luminol
Institutions
Science on StageReview
Bioluminescence has fascinated people for thousands of years and continues to be a popular subject of research, particularly in oceanic exploration projects. The chemical reactions that lead to the production of light by organisms are a great example of biochemistry. The practical activity described in this article is useful for engaging and entertaining students and provoking scientific discussion.
Marie Walsh, Limerick Institute of Technology, Ireland