How global teamwork revealed the mystery at the heart of our galaxy Understand article
This is the story of how scientists created an image of the region around the black hole at the centre of our galaxy by combining many telescopes into one virtual telescope the size of the Earth.
The idea that light could be affected by gravity is actually quite a strange one that was triggered by Einstein’s landmark paper on General Relativity in 1916. He proposed that a mass warps space and time in such a way that light is forced to change direction (figure 1).
This very effect was validated by observations of the solar eclipse in 1919 and is exerted by a force called gravity, which has some special properties.[1] Since then, countless physicists and astronomers have been getting to grips with the mind-bending implications of this discovery.
Image: © NASA’s Cosmic Times
Gravity
Gravity is a very special force; it is the only force that you do not feel when it is the sole force acting. In free fall without air resistance, all experiments behave as if they were carried out in zero gravity, i.e. as if the force of gravity isn’t working. Albert Einstein’s theory of General Relativity describes gravity as the effect of the geometry of space-time on a mass. If space-time is flat because no other mass curves it, then the mass behaves as if no force were acting on it. If space-time is curved by the presence of other masses, then this geometry determines the motion of the mass. Consider two people standing at the Earth’s equator travelling due north. As they travel, along a meridian, they come closer and closer to each other until they meet at the North Pole – not because an attractive force is acting between them, but because the geometry of the earth forces them to do so.
One of the implications of the special properties of gravity is the black hole – an object with such a strong gravitational pull that it warps space-time so intensely that not even light can escape its reach, making observations of them notoriously difficult. Investigating the light emitted or reflected by an object is one of the best ways to learn more about it, and without this source of information many aspects of black holes remain a complete mystery. For example, we do not know what happens in the domain from which no light, and thus no information, can reach us, or how the most massive of them came into existence – some black holes are inexplicably millions or billions of times the mass of our Sun.
Some of these questions will likely forever remain unanswered. However, there is much that can be learned from studying black holes. They are some of the most extreme environments in the universe and places where our models of physics break down. Black holes are extremely small compared to other objects in space with identical masses and so their density is enormous. A black hole with five times the mass of the Sun will hide all its secrets within a sphere with a radius of 15 km.
Seeing the invisible
Due to their nature, observations of black holes have always been indirect, that is, by observing the extreme motion of nearby objects that could only be explained by the presence of a black hole (figure 2). It is similar to how, even though you may be indoors, you can still tell that it’s windy from the swaying trees and flying leaves, despite not being able to see or feel it for yourself.
In this way, astronomers observed stars towards the centre of our galaxy moving extremely fast. Reaching speeds in excess of 7600 km/s, these stars would make the journey from the Earth to the Sun in less than six hours. To keep these stars on their orbits around the centre, an extraordinarily massive object is required to exert the necessary gravitational pull – a black hole with four million times the mass of the Sun. Named Sagittarius A* (Sgr A*) due to its position in the Sagittarius constellation as seen from Earth, we now know it as the supermassive black hole at the centre of the Milky Way. To learn more about Sgr A*, a collective international effort was made to image it, and the Event Horizon Telescope (EHT) collaboration was formed.[2]
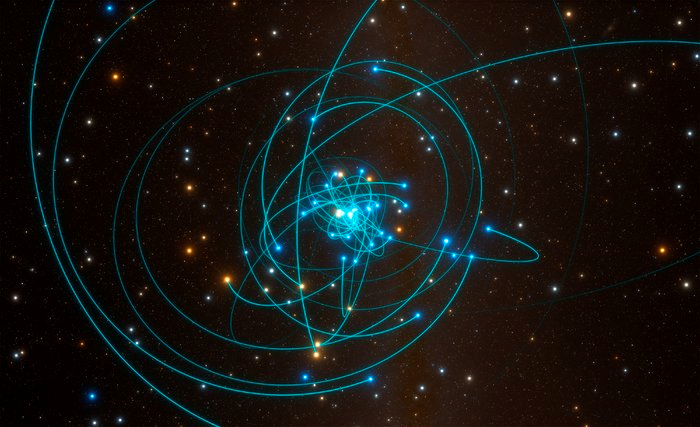
Image: ESO/L. Calçada/spaceengine.org, CC BY 4.0
Imagining the invisible
Since a black hole itself doesn’t emit light, the EHT focused on imaging the surroundings of Sgr A*. Around some of these ultra-compact objects, a disk of dust and gas is formed from which matter spirals into the black hole, crossing the point of no return, or event horizon (figure 3). The inner regions of the disk circle the black hole at immense speeds, which heats them and causes them to glow brightly. This glow originates outside the event horizon and can thus be seen by outside observers. This is what astronomers planned to image by using the aptly named EHT.
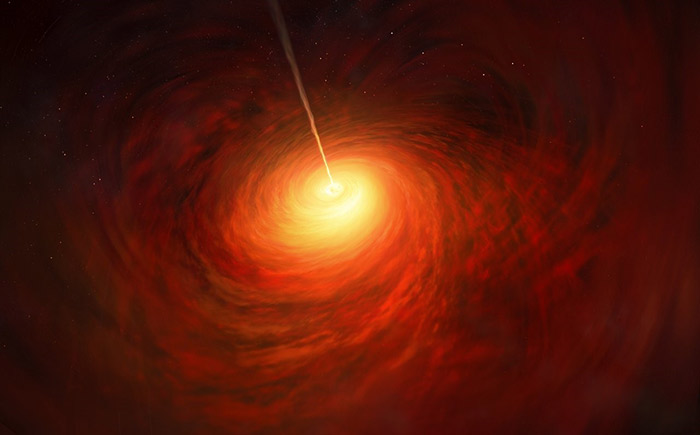
Image: ESO/M. Kornmesser, CC BY 4.0
A major obstacle in observing Sgr A* is that a black hole, even with a mass of four million times the sun, actually appears as a tiny object in our night sky. Taking a picture of it is equivalent to photographing a doughnut on the surface of the Moon. As you can imagine, this would take a telescope with an extremely high power of resolution. The resolution of a telescope is proportional to its diameter, so creating a coherent image would in fact require a telescope the size of the Earth. This was a challenge that required some very innovative methods to overcome.
A telescope the size of the Earth
Astronomers and engineers used a technique known as interferometry to join multiple telescopes across the globe together to observe an object at the same time. This essentially results in a huge virtual telescope – the EHT.[3] The virtual diameter and thus the resolution of the EHT depends on the maximum possible distance between the individual telescopes. However, the light-collecting area of this virtual telescope is far from being fully covered by the real telescopes, so not all the information about Sgr A* can be captured – but a surprising amount of detail can still be obtained. Observing the centre of the Milky Way by using interferometry is somewhat like listening to a song of which only a few notes are played. Even without all of the notes you could still recognise a melody and rhythm, as demonstrated in this video. The more notes you can hear, the more recognisable the tune becomes. As you can imagine, the more telescopes linked into the EHT, the better the image of Sgr A* would be. The use of interferometry has become almost routine in radio astronomy, but for visible light the challenges of this technique are still not feasible. In the wavelength range in which the EHT operates (1.3mm), extremely precise timing of the observations and an immensely powerful computer system to integrate and analyse the data were necessary.
Telescopes at eight locations spread far and wide across the globe and run by various organisations were assembled to form the EHT (figure 4). From Greenland to the South Pole, telescopes were connected to form a virtual telescope that was as big as the Earth and thus was able to construct an image of Sgr A* (figure 5).
Image: NRAO, CC BY 4.0
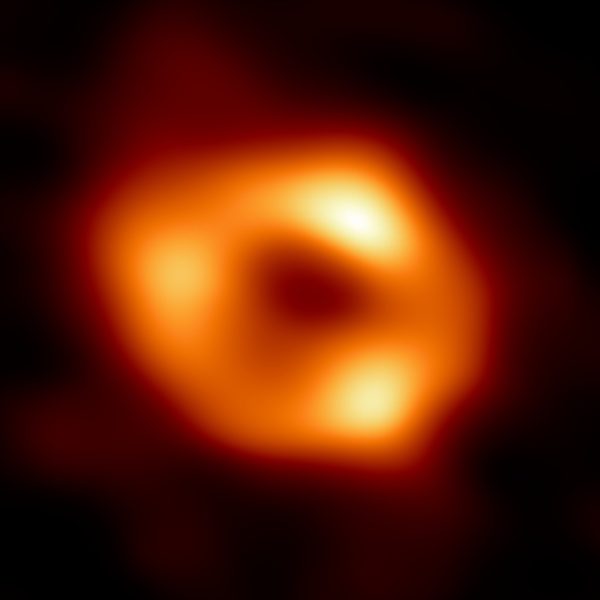
Image: EHT Collaboration, CC BY 4.0
After imaging the central black hole in the galaxy M87 in 2019, the EHT provided the first direct evidence for the presence of a black hole at the centre of our galaxy, and has revealed a new laboratory for testing the limits of our current understanding of physics. The future of this research is incredibly exciting, with the potential for space-based telescopes to join the network. This would create a vastly larger virtual telescope that could image Sgr A* with even greater precision.
References
[1] How the sky looked like during the solar eclipse 1919: https://www.eso.org/public/images/potw1926a/
[2] The Event Horizon Telescope: https://eventhorizontelescope.org
[3] Interferometry: https://www.eso.org/public/teles-instr/technology/interferometry/
Resources
- Get an overview of gravity, general relativity and its peculiarities.
- Read a more in-depth discussion of why gravity is so special, especially in comparison to quantum physics.
- Find out more about the Event Horizon Telescope.
- Learn more about why a photo of a black hole gets better and better if there are more telescopes spread over a larger area.
- Discover how the first image of the black hole at the heart of our galaxy was made.
- Try these activities to understand how we can study very small and large objects that cannot be directly observed or measured: Akhobadze K (2021) Exploring the universe: from very small to very large. Science in School 55.
- Use a digital camera to record parallax shift: Pössel M (2017) Finding the scale of space. Science in School 40: 13–18.
- Learn about gravitational waves and where they come from: Arnaud N (2017) Gravitational waves: a taxonomy. Science in School 41: 40–45.
- Read about the discovery of an entirely new planet type: ESA (2023) Hubble helps discover a new type of planet largely composed of water. Science in School 61.
- Explore the current methods used to discover exoplanets: Vieser W (2020) Hunting for exoplanets. Science in School 49: 8–12.
- Find out the environmental consequences of light pollution: Henshaw C (2022) Too much of a good thing – the problem of light pollution. Science in School 56.
Institutions
Review
The article explains why photographing a black hole was scientifically and technically possible so late. EHT’s idea of using several radio telescopes, spread over a large area of the earth is one of the smartest ideas of this century.
If students ask about black holes, Students ask from the small classes what a black hole means, this article provides support for teachers to explain this concept in a simple way. It could tie into the curriculum topics:
- Physics – optics, gravitational attraction
- Astrophysics – the life of a star, galaxies
Comprehension questions:
- What happens to light when it passes by a celestial body with a very large mass.
- What are the important properties of a black hole.
- Why does a bright area appear around a black hole.
- Why can we only now photograph a black hole.
Corina Lavinia Toma, physics teacher, Romania