Is traditional engineering the right system with which to manipulate our world? Understand article
We are relative newcomers on Earth and still have a lot to learn. Julian Vincent from the University of Bath, UK, investigates some of the lessons we can learn from the living world.
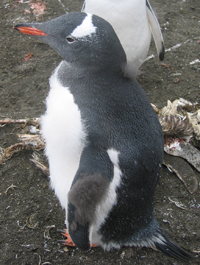
papua)
Image courtesy of Pismire;
image source Wikimedia
Commons
Engineering is probably humankind’s greatest achievement. And like all high-profile activities, it is in danger of becoming our downfall. It’s not that we are too good at it, it’s that we hardly realise that there are alternatives. A new study which compares engineering and the living world as problem-solving systems suggests that we have the tools for sustainable living, but are not using them properly (Vincent et al., 2006). We don’t necessarily have to go down an overtly ‘green’ path, but we need to reorganise current techniques with a different emphasis.
Engineering is a subset of human behaviour. It increases our ability to survive, especially under hostile conditions such as extreme temperatures. Animals and plants are able to survive such conditions because they possess extreme adaptations. For instance, the feathers of the gentoo penguin (Pygoscelis papua) can support a temperature difference of about 60°C across a thickness of 2 cm or so (Dawson et al., 1999); a penguin has a body temperature of 38°C and can survive in temperatures between -30 and -60°C. The desert cockroach (Arenivaga investigata) can harvest water from apparently dry air (O’Donnell, 1982).
However, whereas individual species of animals and plants each have only a few adaptations, and so are found in well-defined habitats, humans can assume a wide variety of survival mechanisms, changing them as the environment changes. Thus, humans are not limited in climatic range, and have overrun the Earth. However, current methods of providing protection or isolation from the climate, such as houses, heating, air conditioning, waterproofing and protection from floods, are energy expensive, accounting for nearly half of our current energy usage.
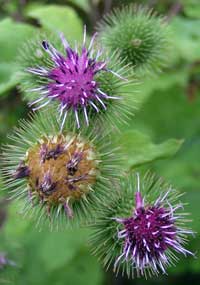
head
Image courtesy of Pethan;
image source: Wikimedia
Commons
Some of these mechanisms are being copied or adapted from nature, and form part of the emerging study of biomimetics (also known as biomimicry, bionics or bio-inspired design). Velcro is the archetype, inspired by the hooked seeds of burdock (Arctium minus) (see images). Superhydrophobicity is another example, driven by observations of the lotus leaf (Nelumbo nucifera), to which no water-based material can stick, and which can be cleaned simply by pouring water over it (see image; Barthlott & Neinhuis, 1997).
Other examples are the non-sticky adhesive hairs on the feet of the gecko (for instance Hemidactylus garnotii), with which it can climb vertical walls (Autumn et al., 2001). Our adhesives would soon become non-sticky with the accumulation of dust but somehow the gecko’s feet clean themselves (Hansen & Autumn, 2005). Other examples are the shapes of trees which, because they mould themselves to prevent any weak points, inspire the design of strong components for use in motor cars; non-reflective surfaces based on the moth-eye and the leaves of plants growing in deep shade, neither of which can afford to lose light by reflecting it. The list continues.
These are all examples of biological engineering: the solution of living organisms to the problems posed by survival – the same problems, essentially, which we have solved using engineering.
In recent years, members of our research group have been comparing strategies to see whether biology has better or different solutions to the problems of survival. To do this, we used a collection of 40 ‘inventive principles’ which Genrich Altshuller, a Russian inventor and thinker, devised to represent the manipulations by which engineers solve problems. He and his colleagues gleaned these by studying successful patents, categorising them in terms of the problem that had been solved and the means of solution. The list forms part of a problem-solving system called TRIZ (Altshuller, 1988).
A problem arises if you want something, but you are prevented from achieving this desire. Plato pointed this out several thousand years ago. Altshuller also realised this, but reformulated and formalised the idea by producing a list of categories that covered all possible results and their conflicts. He then showed that a good invention is successful because it resolves a conflict with a new concept rather than with a compromise that satisfies nobody. Significantly, he listed the ways – the inventive principles – by which the conflict could be manipulated in order to achieve this resolution.
All this was a bit complex for us in our aim to compare biology and technology. Altshuller had help, and together they analysed some 3 million patents. We had less time and fewer people, so we simplified his system, and stated that there are six basic things that we can change in order to resolve a conflict. These are:
- Substance (the material from which something is made)
- Structure (the way the materials are arranged)
- Energy (the source of energy, or the amount of energy, whether more or less)
- Space (space occupied by the system, or space available for it to work)
- Time (the order in which things might happen, or the time required for something to be done)
- Information (the control mechanism and things which influence it).
One of our findings is that biology solves the same types of problem that we solve with engineering, but the method of doing so is similar only 12% of the time. For example, in arthropods the forces in the cuticle, which can come from internal or external sources, are detected by the change in shape of holes placed in the most highly loaded areas and which extend through the cuticle. In engineering, a hole is considered dangerous as it can be the start of a crack, so forces are more likely to be measured by a separate strain gauge. But the arthropod’s cuticular hole is very carefully designed with great attention to detail, is totally safe, and is considerably more sensitive than a strain gauge. We could incorporate such devices, but our engineering rules and preconceptions do not consider such a possibility.
We had a more important result (see graphs). We arranged the solutions according to the size of the system, ranging from nanometres to kilometres and spanning 12 orders of magnitude. We then found even larger differences between technology and biology. In technology, at the micrometre to centimetre level, we solve 70% of problems by manipulating energy in some way. Note that this does not refer to the amount of energy. Examples might be increasing the speed of reaction by increasing temperature, using pneumatic or hydraulic delivery or control of energy, or reducing the energy requirement by allowing a component to resonate.
Nonetheless, when we saw that biology relies on changes in energy no more than 5% of the time, we realised that this is an important difference. In biology, change is effected by imbuing everything with information, starting from the DNA molecule. The proteins that DNA encodes also contain that information and allow the organism to interact with its environment, which represents another source of information. Proteins and their products then interact and ‘self-assemble’ in defined ways to form organelles, tissues, organs and organisms. The ordered arrangement of these levels of organisation, and the behaviour of the resulting organisms, all rely upon their embedded information and reflect it in the patterns that we observe. None of this occurs in the standard methods of engineering, although the idea of self-replicating machines has been around for some time. Adrian Bowyer, a member of our team, is running a project in which the computer programme for making a rapid prototyping machine can be downloaded from the Internet and read by a rapid prototyping machine. This machine will then be able to make a copy not only of itself, but also of (notionally) anything else. More details of this disruptive idea are available from the RepRap websitew1. Nevertheless, all patterns in the manufactured world are the result of conditions imposed by an engineer at some level.
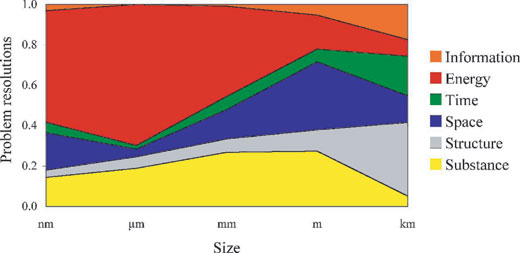
Image courtesy of Julian Vincent
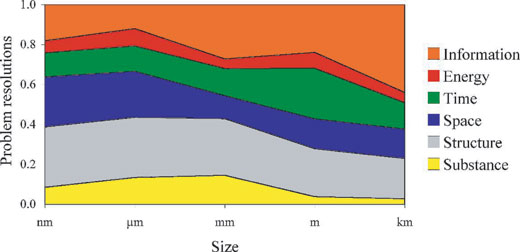
Image courtesy of Julian Vincent
Another difference is that technology is very reliant on materials – for instance, we use over 350 types of polymer in engineering. Biology has just two – protein and polysaccharide. But these are so variable, because of the information they contain, that they can achieve more than any manmade polymer. That information allows them to self-assemble into structures, such as the cell wall of a plant or the cuticle of an insect or lobster, which provide yet more functional versatility. In addition, with only two polymers to deal with, recycling is relatively easy. Current waste reclamation allows us to separate only about six polymers (Table 1) with any reliability. But these six represent most of the functionality we require. Why do we have so many polymers? We should select our materials for their ease of sorting and recycling. Their functionality can be increased even further by copying nature and assembling them into structures such as foams and honeycombs, or combinations and permutations of such structures.
Name | Abbreviation | Stiffness | Use | Comments |
---|---|---|---|---|
Low-density polyethylene | LDPE | Flexible | Films, bags | |
High-density polyethylene | HDPE | Rigid | Films, bottles | |
Polypropylene | PP | Rigid | Films, sheets, bottles | Tough, light, cheap |
Polyethylene terephthalate | PET | Rigid | Films, sheets bottles | |
Polystyrene | PS | Rigid | Films, rigid sheets | Easy to replace |
Polyvinylchloride | PVC | Rigid and flexible | Films, sheets bottles | Easy to replace |
We are only at the beginning of the study of biomimetics and how to make the best use of the technological tricks we can learn from biology, but already we have discovered ways to challenge technology and revealed ways that biological organisms – the great survivors – answer the same problems in a sustainable manner. Now we have to set about changing technology to ensure our survival – although humans have successfully colonised a wider range of environments than any other species, they have done so in a very inefficient way, at enormous environmental cost.
Classroom activity:
A biomimetic challenge
Get your students involved in biomimetics! Here are two challenges from the Science in School editor:
- Can you think of a plant, animal or micro-organism that has solved a problem with which humans still struggle? Do you know what the biological solution to the problem is? How do you think humans might use this?
- Draw or paint a picture of how a plant, animal or micro-organism has evolved to survive in a hostile environment.
Send us your ideas and/or pictures by 30 June 2007, and we will publish our favourite entries together with Professor Vincent’s responses. Don’t forget to tell us your name, age, school and country. Include the text ‘Biomimetics challenge’ in the subject line of your email and send it to: editor@scienceinschool.org
References
- Altshuller GS (1988) Creativity as an exact science. New York, NY, USA: Gordon and Breach
- Autumn K et al. (2001) Evidence for van der Waals adhesion in gecko setae. Proceedings of the National Academy of Sciences USA 99: 12252-12256
- Barthlott W, Neinhuis C (1997) Purity of the sacred lotus, or escape from contamination in biological surfaces. Planta 202: 1-8
- Dawson C et al. (1999) Heat transfer through penguin feathers. Journal of Theoretical Biology 199: 291-295
- Hansen WR, Autumn K (2005) Evidence for self-cleaning in gecko setae. Proceedings of the National Academy of Sciences USA 102: 385-389
- O’Donnell MJ (1982) Hydrophilic cuticle – the basis for water vapour absorption by the desert burrowing cockroach, Arenivaga investigata. Journal of Experimental Biology 99: 43-60
- Vincent JF et al. (2006) Biomimetics: its practice and theory. Journal of the Royal Society Interface 3: 471-482
Web References
- w1 – RepRap website
Resources
- For an example of recent biomimetic research into spider silk, see: Cicognani G, Capellas M (2007) Silken, stretchy and stronger than steel! Science in School 4 : 15-17.
Review
By reading this article you will learn some new synonymous terms: biomimetics, biomimicry, bionics and bioinspired design. They are all words describing how living creatures are used as models or inspiration for engineers when developing new materials or constructions. Animals and plants are very well adapted to their environment because they have solved challenges related to their survival, for example isolation from extreme heat or cold. They have evolved materials and strategies which are very well suited for certain aspects of their life, such as reproduction, feeding, protection and so forth. Professor Vincent gives us examples of so-called biological tricks that can be useful in engineering. By comparing basic elements within biology and technology, scientists and engineers will achieve a better understanding for resolving their problems. There are plenty of things we can learn from nature!
Don’t forget to study and involve your students in the two challenges at the end of the article!
Sølve Marie Tegner Stenmark, Norway