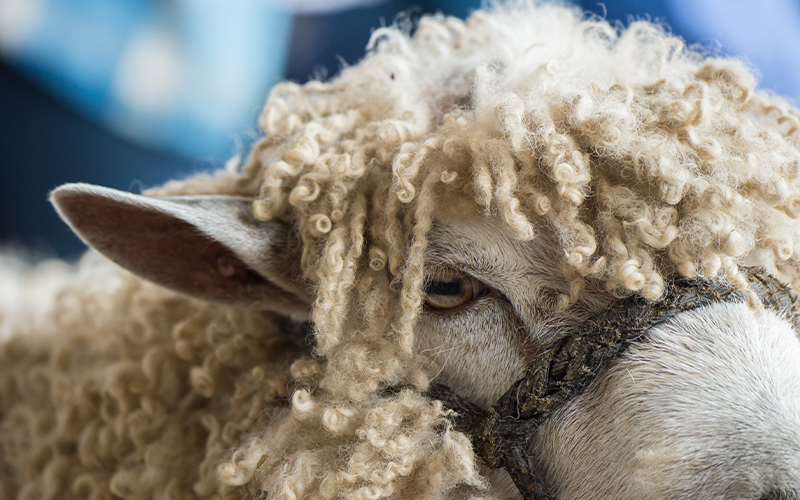
Extract value from wool waste: keratin and the circular economy
Spinning a yarn: explore the chemistry of wool and use it as a raw material for biobased products through simple hand-on activities.
Camelids are famously robust and useful animals. Surprisingly, their unusual antibodies are just as sturdy and are now revolutionizing medical science.
The year 2024 was designated the United Nations International Year of Camelids. Camelid animals, including alpacas, camels, and llamas, play important roles as a means of transport and a source of milk, meat, and fibre, even in the most extreme environments. Perhaps you have patted the soft wool of an alpaca in the zoo or even taken a swingy ride on a camel on your holidays. But did you know that these long-legged, long-necked, serene-looking hairy creatures have also made important contributions to advance science?
Conventional antibodies are Y-shaped proteins produced by the immune cells (B cells) of mammals like mice, dogs, and humans. The biological function of antibodies is to specifically bind to foreign proteins from microbes, such as viruses, bacteria, and parasites, and thus give protection against infections. However, antibodies are also important research tools and are even used as medicines to treat disease, since it is possible to develop antibodies that specifically bind to any target of choice.
Conventional antibodies are Y-shaped proteins composed of four protein chains: two identical heavy and two identical light chains (Figure 2). These chains are composed of two (light) or four (heavy) globular protein domains (turquoise shapes in Figure 2). The upper parts of the light and heavy chains together form the binding region, which is like a ‘hand’ that can grab the target. Depending on the shape of this hand, a certain antibody can only grab a certain shape. In other words, antibodies engage in specific recognition, uniquely recognizing their target and binding to it like molecular velcro.
Over three decades ago, a group of Belgian researchers surprised the scientific community with their finding that camelids also produce a smaller version of antibodies.[1] These antibodies are composed of only two heavy chains and have no light chains. Scientists then discovered that they could separately produce the hand domain of these ‘heavy-chain-only’ camelid antibodies to make an even smaller version,[2] which are known as nanobodies (Figure 2).[3]
These little nanobody hands can bind their target just as strongly and precisely as antibodies. Compared with conventional antibodies, nanobodies are more stable, can function in hot, acidic, or alkaline conditions, and can’t easily be degraded.[3] Because of their small size (2.5 × 4.0 × 3.0 nm),[2] nanobodies can easily sneak into places that the much larger antibodies cannot access. One of the ‘fingers’ that nanobodies use for target recognition is sometimes longer than those found in conventional antibodies and is thus able to get into deep pockets on other proteins. Nanobodies are water-soluble, which is an important property in both research and clinical applications. Their ability to be transported in the bloodstream helps their distribution throughout the body. Finally, because they are small, unbound nanobodies are rapidly filtered out of the bloodstream by the kidneys, which reduces the number of potential side-effects. Overall, it seems that nanobodies have much in common with their source: camelids that are able to thrive in deserts where the conditions are some of the harshest on earth! The unique properties of nanobodies make them potentially useful for a variety of applications, both in research and in medicine.
Nanobodies have become useful tools in research. A particularly common use of nanobodies is in the field of structural biology, a branch of research where people investigate the structure of biomolecules like proteins. One very useful method to determine protein structures is called X-ray crystallography, which involves bombarding protein crystals with X-rays. This technique requires regular crystals, in which all the protein molecules need to be packed in an orderly fashion. Growing these crystals is often a challenging task because many proteins are flexible and can take on slightly different forms (aka conformations) and thus fail to form regular crystals. A nanobody can be used as a ‘chaperone’ to lock the target protein into a specific form and help the formation of protein crystals (see Figure 4).[4]
The very first nanobody medicine, called Cablivi, was approved in 2018 to treat a rare, life-threatening blood clotting disorder called acquired thrombotic thrombocytopenic purpura (aTTP). In aTTP, a protein called von Willebrandt factor forms large clumps when interacting with blood platelets, which leads to severe blood clotting. The Cablivi nanobody was designed to bind the von Willebrandt factor and block its dangerous interaction with platelets. It is now being used in hospitals to treat this deadly disease.[5] A newer medicine, Ozoralizumab, used in Japan, binds and blocks an inflammation-causing human protein called tumour necrosis factor alpha (TNFα), which is a real troublemaker in the joints of people with rheumatoid arthritis.[6] Another nanobody medicine, Envafolimab, binds T cells and activates them to attack cancer cells.[7]
As mentioned, nanobodies have several uses in research and in medical applications. However, the coolest thing about nanobodies is that they can also be easily attached to other molecules. We can think of nanobodies as building blocks that can be attached to functional particles to guide them to specific places in a body or sample. These particles might a) serve as a marker to allow the detection/localization of the target or b) deliver an ‘effector’ function like a medicine (Figure 5).
Radioactive compounds linked to nanobodies can serve both as both detectors and destroyers of cancer cells. Radioactive atoms can be attached to nanobodies so that they can be detected in a living organism, for example, human patients. These radioactive atoms emit radiation that passes easily through tissues and can be detected using positron emission tomography (PET). In nanobody-guided PET imaging with gallium, the irradiation dose is extremely low (0.013 mSv);[8] in comparison, according to the United Nations Scientific Committee on the Effects of Atomic Radiation (UNSCEAR) 2008 Report[9], the yearly average dose of irradiation in our everyday lives is 2.4 mSv. Tumour-specific nanobodies linked to the minimally radioactive compound gallium 68 were found to be useful for imaging colorectal tumours and cancer cells that have spread to other parts of the body in patients,[7] which is an example of a modified nanobody serving as a detector. At higher doses, radiation can also be used to kill cancer cells, so cancer-cell-specific nanobodies linked to radioactive atoms can directly deliver radiation to the tumour, leading to its destruction. In one study, radioactive lutetium 177 atoms, linked to a breast-cancer-specific nanobody, delivered a tumour-targeted toxic irradiation dose of 900 mSv.[10] By targeting the radiation to the tumour, we can minimize damage to healthy cells and thus reduce side effects.
The nanobody trend, which our friends the camelids started a couple of decades ago, has advanced in gigantic leaps in recent years. It is now possible to develop nanobodies in any molecular biology laboratory without the need for a herd of llamas! Nanobodies can now be created fully synthetically, with the help of simple microorganisms, namely bacteria, yeast cells, and viruses. When the COVID pandemic hit, it took colleagues at European Molecular Biology Laboratory (EMBL) less than three weeks to develop synthetic nanobodies that efficiently bound the coronavirus.[11] However, identifying efficient nanobodies is just the first step; to make it to the clinics, nanobody-based medicines, just like any other medical product, must go through vigorous pre-clinical animal testing and clinical studies in human patients to ensure their safety and efficacy. There are now even efforts to identify nanobody binders by using artificial intelligence,[12] an approach that may further streamline the production of these multifunctional proteins. Unfortunately, synthetic nanobodies often don’t perform as well as those derived from immunized camelids and need further adjustment for optimal activity. There is still much to learn!
Overall, nanobodies are versatile tools that are easily modified and connected to other molecules, and modified nanobodies can help researchers and patients in many ways. Since their discovery, they have been an inspiration for researchers around the world to develop innovative solutions. Maybe one day YOU will come up with a research or treatment idea for which a nanobody, this magnificent molecular velcro, will be exactly what you need.
[1] Hamers-Casterman C et al. (1993) Naturally occurring antibodies devoid of light chains. Nature 363: 446–448. doi: 10.1038/363446a0
[2] Kunz S et al. (2023) NANOBODY® molecule, a Giga medical tool in nanodimensions. International Journal of Molecular Sciences 24: 13229. doi: 10.3390/ijms241713229
[3] Jin BK et al. (2023) NANOBODIES®: A review of diagnostic and therapeutic applications. International Journal of Molecular Sciences 24: 5994. doi: 10.3390/ijms24065994
[4] Cheloha RW et al. (2020) Exploring cellular biochemistry with nanobodies. The Journal of Biological Chemistry 295: 15307–15327. doi: 10.1074/jbc.REV120.012960
[5] Canadian Agency for Drugs and Technologies in Health (2022) Executive Summary. Clinical Review Report: Caplacizumab (Cablivi). Indication: Indicated for the treatment of adults with acquired thrombotic thrombocytopenic purpura (aTTP) in combination with plasma exchange (PEX) and immunosuppressive therapy. Available from: https://www.ncbi.nlm.nih.gov/books/NBK567591/
[6] Tsumoto K, Takeuchi T (2024). Next-generation anti-TNFα agents: The example of ozoralizumab. BioDrugs: Clinical Immunotherapeutics, Biopharmaceuticals and Gene Therapy 38: 341–351. doi: 10.1007/s40259-024-00648-3
[7] Cui C et al. (2024). Model-informed drug development of envafolimab, a subcutaneously injectable PD-L1 antibody, in patients with advanced solid tumours. The Oncologist 29: e1189–e1200. doi: 10.1093/oncolo/oyae102
[8] Li Let al. (2023) Immuno-PET of colorectal cancer with a CEA-targeted [68 Ga]Ga-nanobody: from bench to bedside. European Journal of Nuclear Medicine and Molecular Imaging 50: 3735–3749. doi: 10.1007/s00259-023-06313-1
[9] The United Nations scientific committee on the effects of atomic radiation (2008) Sources of ionizing radiation. UN Publications. ISBN: 978-92-1-142274-0
[10] D’Huyvetter M et al. (2014) Targeted radionuclide therapy with a 177Lu-labeled anti-HER2 nanobody. Theranostics 4: 708–720. doi: 10.7150/thno.8156
[11] Custódio TF et al. (2020) Selection, biophysical and structural analysis of synthetic nanobodies that effectively neutralize SARS-CoV-2. Nature Communications 11: 5588. doi: 10.1038/s41467-020-19204-y
[12] Bennett NR et al. (2024). Atomically accurate de novo design of single-domain antibodies. bioRxiv: the preprint server for biology. doi: 10.1101/2024.03.14.585103
This article offers a clear introduction to nanobodies, a cutting-edge topic in immunology and biotechnology, tailored for students aged 11-14. It aligns with key biology curriculum topics like the immune system and advances in medical treatments.
Science Teachers can use the article to encourage discussions on the future of medicine and ethical considerations in biotechnology. It also serves as a useful comprehension exercise, helping students summarize and explain key concepts while developing critical thinking skills.
António Pedro Fonseca, Science Teacher and Ph.D. Researcher, Portugal
Spinning a yarn: explore the chemistry of wool and use it as a raw material for biobased products through simple hand-on activities.
Why was a Nobel prize awarded for 'click chemistry'? Learn about the ground-breaking advance behind this simple-sounding…
How can AI systems like those developed to beat humans at games help unlock the secrets of protein…