Hydrocarbons: a fossil but not (yet) extinct Understand article
Continuing our energy series, Menno van Dijk introduces us to the past, present and future of hydrocarbons – still the most common of all fuels.
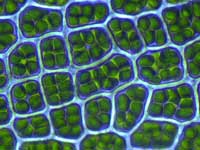
leaf (Mnium hornum); the parts
of the plant cells which store
light energy as molecular fuel
(chloroplasts) are clearly visible
Image courtesy of Kristian Peters
and Hans Ferdinand Maßmann;
image source: Wikimedia Commons
While researchers today are working on the development of clean, renewable fuels, our society is still almost entirely dependent on fossil fuels. How are they formed, how much is there of them, and how long will they last?
Hundreds of millions of years ago, the world was a wilderness, but not void. A diversity of animals and plants populated the landmass. The seas bubbled with life and, like today, the largest part of the biomass consisted of microscopically small organisms. They all extracted the energy for their organic molecules either directly or indirectly from sunlight.
Storage in reservoirs
Since then, the continents have drifted apart, with some landmasses disappearing into the depths and others being heaved up. Wind, ice and rain caused erosion on land, which created great masses of sediment, especially in river estuaries. Some sediments were porous (such as sand or the skeletons of calcareous animals), others impermeable (such as clay). Organic material was buried, too, and the vertical movements of landmasses could take it several kilometres below the ground. There, it was warmed up by heat from the interior of Earth.
The high temperature and high pressure at that depth caused the organic material to break down and be converted to a fluid with a diverse collection of chemical structures: volatile hydrocarbons such as methane and ethane, short and long paraffinic molecules, aromatics, and highly complex and large polycyclic structures. Since they all consist mainly of carbon (C) and hydrogen (H), they are collectively called ‘hydrocarbons’.
This fluid, mostly together with water that was also trapped, worked its way up through porous rock until it was – in some cases – stopped by an impermeable layer of sediments. Where the geometry, determined by breaks and deformations, had formed three-dimensional enclosures, or reservoirs, the fluid accumulated and remained there to simmer quietly.
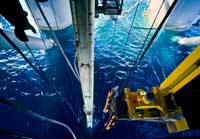
Image courtesy of Mayumi Terao
/ iStockphoto
Due to the absence of oxygen, the solar energy stored as chemical energy in the molecules was not burned up (oxygenated), but preserved for millions of years. Sometimes a separate gas phase was formed above the oil, and on other occasions, when only very small hydrocarbon molecules found their way to a reservoir, a ‘gas bubble’ formed. Note that such a gas bubble, just as an oil reservoir, is enclosed in porous rock, the pores of which are filled with oil and/or gas and/or water.
On land, organic material such as trees and plants got buried, too. Under favourable conditions, when they were quickly covered by sediment and thus sheltered from oxygen, preventing rot, these were converted to thick layers of coal. In some places, the hydrocarbons came into contact with bacteria with an appetite for certain molecules, which changed their composition. So each reservoir storing accumulated solar energy will have its own character in terms of reservoir shape and hydrocarbon composition.
Energy hunger in the modern world
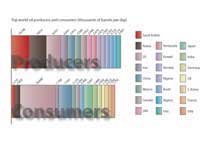
consumers (thousands of barrels
per day). Click to enlarge image
Image courtesy of Vienna Leigh
Over time, we humans have developed a great hunger for energy. Originally, this was fulfilled by firewood. When the growing of wood couldn’t keep up with the growing demand for energy, we started digging up million-year-old firewood from coal mines. But the solid form of coal was cumbersome, dangerous to dig up and not very economical. Eventually, oil reservoirs were discovered. While man’s use of oil dates to prehistory, the first modern oil well was drilled in the United States on 27 August 1859 by Edwin Drake in Pennsylvania. Later, wells were drilled especially in the Middle East, where vast resources of fairly easily extractable oil appeared to be situated under the sand.
The combustion engine caused an explosive growth in the demand for oil. This grew so quickly that the end of the supply seemed near. The Club of Rome, a global think tank for political issues, warned that mankind was quickly running out of energy reserves. So people started to search in more inaccessible places and discovered much more oil under water: the North Sea, the Gulf of Mexico, the Niger Delta in Nigeria. Driven by high oil prices, technology was developed to recover oil from increasingly deep water. Moreover, natural gas came into view. Originally, striking gas on a drill was considered bad luck. Locally, you may have been able to use it, but exporting it over long distances was far too expensive. Here too, technological developments changed the situation.
Liquefied gas
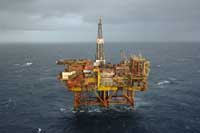
the North Sea
Image courtesy of the Shell Image
Library
With liquefied natural gas technology, natural gas is compressed to about 1/600th the volume, and became transportable over long distances. Recently, it also became possible to chemically transform gas on a commercial scale to heavier (liquid) hydrocarbons such as gasoline or diesel fuel (GtL, gas to liquid). Thus, huge gas reserves (in the Persian Gulf, for example, there is a reservoir 10 times as large as Slochteren in the Netherlands, the largest European natural gas field, estimated at 1.5 × 1012 m3; Russia also holds vast gas reserves) could be used to satisfy the hunger for energy.
All this was possible thanks to the development of highly advanced technology. The first oil well for Shell in Malaysia, in Miri, was only 140 m deep, and was drilled in 1910 with a technique that the Chinese had used for centuries to drill for salt. In the 60 years of its existence, 100 000 m3 of oil were retrieved by a pumpjack.
Today, oil is extracted from reserves in up to 2.5 km deep water, 6 km below the seabed. This requires the precise drilling of a 6 km deep, 50 cm diameter hole from a drilling platform bobbing a few kilometres higher up on the sea. Then, at the bottom of the sea, a construction called a subsea well head has to be placed on the well, from which the oil must flow to a production platform which sometimes lies dozens of kilometres away. This is not to be confused with the drilling platform, which drills and constructs the wells and then goes away. Production platforms instead contain the processing equipment.
Between the reservoir and the platform, a multitude of problems can occur which must be known and mastered. Besides knowhow and technology, this requires a lot of money, and this type of oil field can only be profitable if it produces large quantities of oil per day: off the coast of Malaysia, a new field has recently been found, which will produce in four days as much as the first source in Miri produced in 60 years: that’s 650 000 barrels of oil, about 100 000 m3.
Hydrocarbon facts
Estimated R/P: up to 93 years
Cost per kWh: The cost depends enormously on the source. Production costs range from a handful of dollars per barrel for easy oil in Saudi Arabia to tens of dollars per barrel for heavy oil in remote locations.
Risks: Pollution during transport and production; CO2 production when used
Estimated research time: Research will be done for as long as these fuels exist, to make them cheaper, cleaner and more energy efficient, to extract a greater fraction of oil from a reservoir (e.g. by enhanced oil recovery), and to be able to economically exploit small reservoirs, too.
Where to go from here?
One thing is clear: all the oil or gas that has been pumped out is gone forever. Slochteren is running out, the famous North Sea fields are running out, and even in the Gulf of Mexico, the most important oil source for the most energy-hungry country in the world – the USA – the fields being found are increasingly small. Will the hydrocarbon economy soon die a slow, or perhaps a quick, death? Ultimately, supplies are certainly finite, but there are still a few aces up the hydrocarbon sleeve.
Enhanced oil recovery
Depending on the precise circumstances of an oil reservoir, approximately one-third of the available oil is generally extracted. The rest stays behind in the pores of the rock. Using technology, something can still be done to extract more oil: from relatively simple water injection to press the oil from the reservoir, to sweeps with surfactants and polymers to loosen some of the oil from the rock. Thanks to high oil prices, these enhanced oil recovery techniques are becoming very interesting.
Heavy oils and oil sands
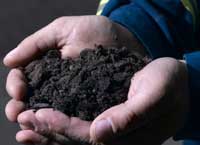
sand recovered in Fort McMurray,
Alberta, Canada
Image courtesy of Rob Belknap /
iStockphoto
There are also reservoirs which contain very heavy, i.e. viscous, oil. Previously, these were not economically developed, but here too, technology can bring about change. But it is not easy and will require large investments, also in knowledge building.
Hyperheavy oil is still in abundance. In oil sands and oil shale, reserves of hydrocarbons are stored – the size of many times the amount of ‘easy’ oil. In Canada, 200 000 m3 of oil are produced daily from excavated tar sands. This is a costly and difficult process, if only because of the extremely low temperatures that prevail in that region. Moreover, the process of producing useful fluids from oil sands requires quite a bit of energy and is not so efficient. For the deeper stocks (80% of the total), no recovery method is yet available.
Coal
Coal reserves are still in abundance, too. In recent years, coal has been the fastest growing energy source. This is because of the growing economy – and consequent demand for energy – in China, which itself has only limited oil and gas reserves, but a lot of coal. China consumes about 40% of the global coal production. But this is not without its price: the deaths of dozens of miners per day (!) and huge environmental damage (by acid rain, among other causes) are associated with the burning of coal. With modern processes (such as transformation of coal to gas) it is possible to produce energy from coal in a less environmentally damaging way. And with more money and less haste, the safety situation can also be improved.
Gas hydrates
Methane, of bacterial or fossil origin, which is released at the bottom of the sea, can form what are known as gas hydrates. This is a kind of ice which, due to methane trapped inside (under pressure), has a higher melting point. Great quantities of methane are likely to be stored in such huge hydrate fields at the bottom of the ocean, including the east coast of the United States. The total energy resources in gas hydrates on Earth are estimated to be greater than those of all other known fossil fuels combined – ever. However, people don’t have even the slightest idea yet of how to exploit these reserves (safely).
For a science fiction outlook on what might happen if these hydrate fields were to be destabilised, see Schätzing (2004).
Reserves and production
Large parts of Earth have been examined for the presence of oil and gas fields. Most hydrocarbon regions have been found: those are the known oil and gas regions, including our own North Sea. Within these areas, reservoirs are still being found, but the really big ones are known, and it is becoming increasingly difficult and costly to develop the new ones. The table below gives an overview of the current proven reserves. There is considerable uncertainty in these numbers, though, both of a technical (estimating the size of a reservoir is very difficult) and of a political nature (countries and companies can have all sorts of reasons to make the reserves look larger or smaller).
1.7 x 1022
Reserves | Annual production | ||||
---|---|---|---|---|---|
m3 | Joules | m3 | Joules | ||
Crude oil | 1.9 x 1011 | 6.8 x 1021 | 4.7 x 109 | 1.7 x 1020 | 41 |
Natural gas | 1.8 x 1014 | 6.4 x 1021 | 2.7 x 1012 | 9.6 x 1019 | 67 |
Coal | 8.0 x 1017 | 2.9 x 1015 | 6.1 x 1019 | 277 | |
TOTAL | 3.0 x 1022 | 2.9 x 1015 | 3.3 x 1020 | 93 |
Global proven reserves and annual production of hydrocarbons in terms of volume (m3 gas under standard condition of 1 bar, 15 °C) and in terms of energy content. R/P is the ratio of reserve to annual production, giving the amount of years the known reserves will still last if exploited at the current production rate
Data source: BP statistical review (www.bp.com)
According to this table, at the current consumption level we would still have 93 years ahead of us if we were to use all fossil energy. That sounds more reassuring than it is, for several reasons:
- There is a large uncertainty in the number 93. It doesn’t mean that after exactly 93 years of wasting energy, time will suddenly be up – we are already starting to feel the effects of the fossil fuels running out.
- Processes to retrieve heavier oils also require more energy, so less energy will effectively be at our disposal from these than from ‘easy’ oils.
- When the energy consumption, and consequently its production, grows, the number of years we can still use the reserves decreases (R/P).
- New projects that could add reserves require huge investments and take a long time from concept to production. A simple oil well costs around €1 million, but a deep-water well may be more than €100 million. A small production platform costs €1 billion. In the Athabasca oil sands project in Canada, $45 billion has already been invested, and there are still $50 billion to come by 2012. Between the discovery of a reservoir and the first production, eight years can easily pass. So shareholders and politicians have to supply time and money, but they generally have little patience. If there is no short-term reason to invest, we will therefore create a long-term energy shortage.
- For many sites, poorly predictable political complications play a role. Therefore, it may not be possible to access the resources.
There are many factors which play a role in finding and producing fossil fuels, but the main ones are that the amount of hydrocarbons is finite and that the proven reserves should not be seen as a reserve barrel that we can open at any moment: a lot of time and money has to be invested before they can be used. It is conceivable that there will be production problems before the lack of reserves becomes problematic.
Hubbert peak
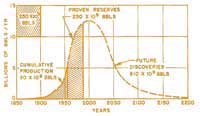
by Hubbert in 1956.
Click to enlarge image
Public domain image; image source:
Wikimedia Commons
Based on an analysis of the cycle of discovery, development, investment in infrastructure, more discoveries, and then slowly running out of reserves, Marion King Hubbert, a geoscientist who worked at the Shell research lab in Houston, Texas, developed a method of analysis which successfully predicted the peak production rate of oil on the American continent for 1970. He made this prediction in 1956. His theory can be applied to an individual oil field or a whole a country, and also worldwide. Applying this method to our planet, we should have reached this peak point by now. If so, we have already passed the peak oil production per capita.
Finally, it should be noted that hydrocarbons are not only used for energy. Approximately one-tenth is used in the (petro)chemical industry to produce a huge range of products: from plastics to solvents, drugs and detergents. Just like energy, these have become an essential part of our lives. In short, fortunately, there are still fossil fuels, but concerning the R/P, we should not think of it as Reasonably Problem-free but as ResPite!
Tankers and pipelines
The technology of oil transportation has evolved alongside the oil industry. Break-bulk boats and barges were originally used to transport oil in wooden barrels. But such barrels were heavy, leaky, and expensive, accounting for up to half the cost of petroleum production. In 1876, Ludvig and Robert Nobel, brothers of Alfred Nobel, founded Branobel in Baku, Azerbaijan, one of the largest oil companies in the world during the late nineteenth century. They pioneered successful oil tanker engineering, but also experienced some of the first tanker disasters. Today’s supertankers are up to 400 m long, with a capacity of up to 500 000 DWT (deadweight tonnage, a measure of how much weight of cargo a ship can safely carry). They can transport two million barrels of oil, which corresponds to the oil consumption of France per day in 2007.
A new tanker of 250 000-280 000 DWT cost US$116 million in 2005 – but oil tankers are often sold second-hand. As of 2007, the CIA statistics counted 4295 oil tankers of 1000 DWT or greater worldwide: this amounts to about 37% of the world’s fleet in terms of DWT. In 2005, 2.42 billion tonnes of oil were shipped by tanker: 76.7% of this was crude oil, the rest consisted of refined petroleum products. With the exception of the pipeline, the tanker is the most cost-effective way to move oil today.
By the sheer amount of oil carried, modern oil tankers have to be considered a threat to the environment, with oil spills having devastating effects. Crude oil contains polycyclic aromatic hydrocarbons which are very difficult to clean up, and last for years in the sediment and marine environment. Marine species constantly exposed to them can exhibit developmental problems, susceptibility to disease, and abnormal reproductive cycles. The International Tanker Owners Pollution Federation has tracked 9351 accidental spills that have occurred between 1974 and 2008. Most spills result from routine operations such as loading and discharging cargo, and taking on fuel oil. While over 90% of the operational oil spills are small, resulting in less than seven tonnes per spill, spills resulting from accidents like collisions, groundings, hull failures, and explosions are much larger, with 84% of these involving losses of more than 700 tonnes.
Modern pipelines have existed since 1860, and today span the world in a growing network millions of kilometres long, with Russia and the rest of Europe each contributing about 250 000 km of gas and oil pipelines (70% of these transport gas). They generally constitute the most economical way to transport large quantities of oil or natural gas over land. Oil pipelines are made from steel or plastic tubes with inner diameters ranging from 10-120 cm, and are typically buried 1-2 m deep. The oil flow of about 1-6 m/s is ensured by pump stations along the pipeline. Multi-product pipelines are used to transport two or more different products in sequence in the same pipeline. There is usually no physical separation between the different products, so some mixing occurs, producing polluted interface which is removed from the pipeline at the receiving facilities. For natural gas, pipelines are constructed of carbon steel and vary from 5-150 cm in diameter, depending on the type of pipeline. The gas is pressurised by compressor stations and is odourless unless mixed with a mercaptan odorant, where required by a regulating authority.
Gas and oil pipelines are not merely an element of trade: they connect to issues of geopolitics and international security, and their construction, placement, and control often figure prominently in state interests and actions. Pipelines cross areas prone to earthquakes and wars, nature reserves and the bottom of seas. As they contain flammable or explosive material, they pose special safety concerns, with ruptures and deadly explosions being the most common accidents.
Acknowledgments
The article was originally published in Dutch:
van Dijk M (2007) Koolwaterstoffen: fossiel maar (nog) niet uitgestorven. Nederlands Tijdschrift voor Naturkunde 73 (7): 206-209
and has been republished online at www.kennislink.nl. It has been translated and adapted for Science in School with the help of Roland van Kerschaver, Jos de Graaf and Salvatore Accardi.
References
Review
The technology may be dinosaurial, but fossil-fuel burning continues to be an integral and increasingly costly part of our modern way of living, not least because of the rapid industrialisation of the most populous nations on Earth.
Along with pharmaceuticals, oil is big business with massive science input. Van Dijk’s article can give science teachers a route into discussing some of the following issues with their classes. Students could consider the economics of how even relatively inaccessible fossil fuel reserves are worth extracting today. How much will a barrel of oil have to cost before exploiting the ‘hyperheavy’ reserves described is worth the hassle? Students could look at how the distribution of hydrocarbon reserves across our planet has affected international relations, from both strategic and economic perspectives.
And while the non-renewable nature of this resource is well known to most students, the fact that around one-tenth isn’t used as fuel at all is less appreciated. What arguments could the students develop to reduce consumption of fossil fuels to secure reserves of fossil feedstock for uses including ‘plastics, solvents, drugs and detergents’? The energy question will require a host of answers, and today’s science students may develop some of the solutions, as well as being made to live with a smaller hydrocarbon footprint in the future.
Ian Francis, UK