Finding the recipe for life on Earth Understand article
Three key factors were required for life to develop on Earth – but which factor came first? Recent research could help settle the debate.
Stop for a moment to consider yourself: think about your eyes reading this, your brain absorbing it, and your body containing and powering these organs. Ask yourself: how did this complex, living biochemical machinery come to be? It’s a profound question that has driven scientists for centuries. Charles Darwin partially answered it with his theory of evolution, detailing how each species emerged from a previous one.
If you trace evolution back far enough, there must have been a time when cells themselves first came to exist – the origin of life, which happened over 4 billion years ago. Today, researchers are trying to solve the puzzle of exactly how this happened, but as it was so long ago, that’s very hard.
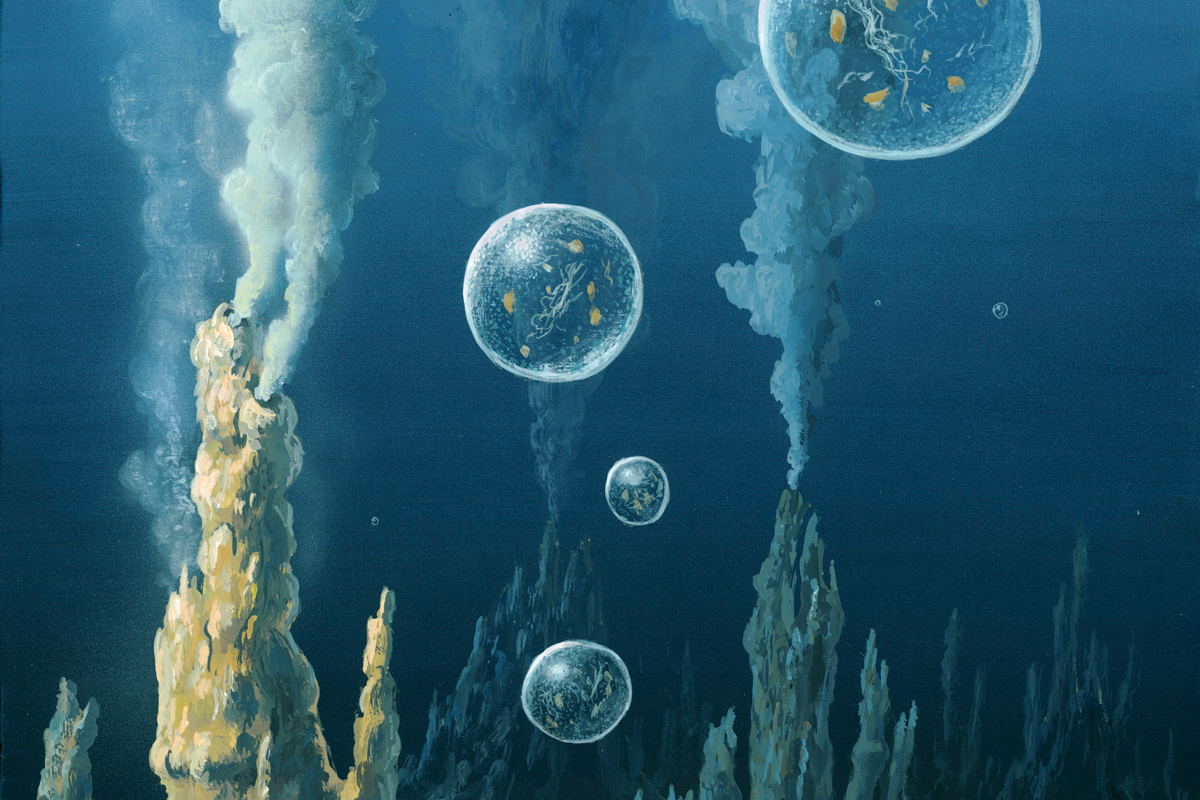
Richard Bizley/Science Photo Library
Comparing modern life forms on Earth provides a powerful argument for how life originated. We humans are more similar to trees, insects, bacteria and all other life than it may seem at first, in three important ways. First, all species use similar molecules to pass on genetic information: ribonucleic acid (RNA) and deoxyribonucleic acid (DNA). Second, different species’ cells look roughly similar – even single-celled organisms share characteristics with the cells of larger organisms. And third, the way all cells get the energy they need for their biochemical processes through metabolism is very similar. This argument leads to the idea that three key factors were required for life to develop on Earth: nucleic acids (RNA and/or DNA); a membrane, compartment or some way to contain the cell’s contents; and metabolism.
Researchers continually offer new ideas on how life might have originated, but a big cause of arguments among them has been the question of which of the three key factors emerged first. More recently, however, a new idea has emerged that could settle that argument: what if all three appeared at the same time?
Early Earth experiments
We have known for a while that organic molecules can be produced under the type of conditions that might have been found on the early Earth. The most famous first step in understanding how life emerged is Stanley Miller and Harold Urey’s 1952 experiment (Miller, 1953). Firing electrical sparks into flasks containing water, ammonia, methane and hydrogen produced some organic substances, including amino acids. However, this failed to explain many aspects of life’s emergence, because it didn’t produce other important biological molecules, such as nucleic acids.
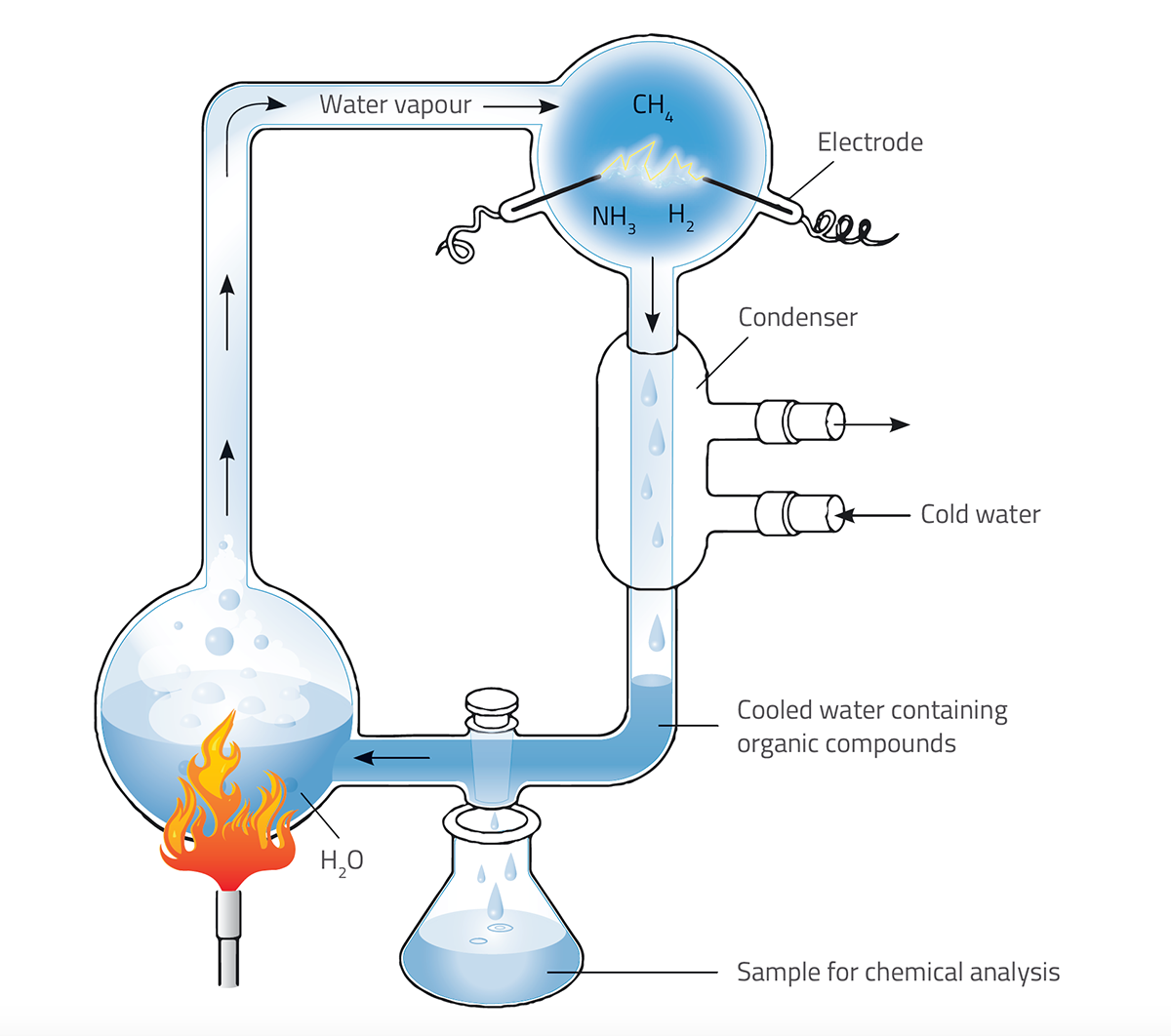
Nicola Graf
In the early 1980s, Tom Cech and Sidney Altman made the Nobel prize-winning discovery of enzymes made of RNA, called ribozymes. Because RNA can encode genetic information and ribozymes can manipulate it, scientists suggested that RNA enabled life by itself, before the other factors were in place. This idea is known as the ‘RNA-first’ hypothesis.
John Sutherland, who now works at the Medical Research Council (MRC) Laboratory of Molecular Biology in Cambridge, UK, is among those who found evidence apparently supporting an RNA-first case. In 2009, his team showed how a ribonucleotide could form under conditions that might have existed on the early Earth (Powner et al., 2009).
Sutherland’s team then sought a better way to make ribose sugars. Researchers had shown that they could be made from formaldehyde, but this route was “really messy and difficult”, Sutherland observes. His team explored an alternative route using just hydrogen cyanide, hydrogen sulfide and ultraviolet light. As well as making the ribose sugars, they produced over 50 different molecules, including some that could help make amino acids – which could assemble to make the proteins that form the physical structure and enzyme machinery of living cells. Making sugars and amino acids together is an important advance, as today’s cells break down sugars in metabolism to get the energy they need (Ritson & Sutherland, 2013).
In 2015, the MRC team showed that the same chemical system leads to fatty molecules that can form cells’ outer walls, defining their shape (Patel et al., 2015). And the system also has similarities to metabolism itself. “It’s consuming small molecules and making bigger molecules”, Sutherland says. “It does suggest that all the subsystems could have emerged at the same time.”
Following on from this, Sutherland and his colleagues published a study in 2019 suggesting that RNA and DNA might have appeared at the same time, thus further challenging the RNA-first hypothesis (Xu et al., 2019).
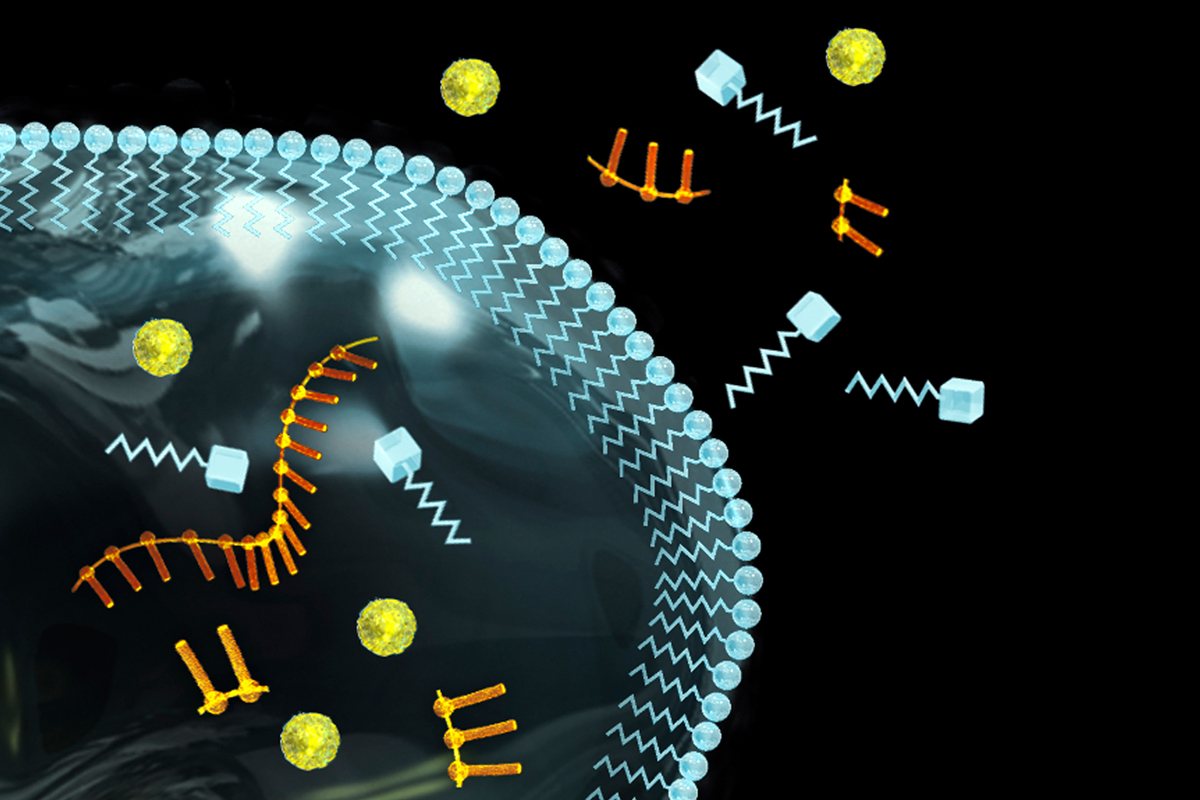
Henning Dalhoff/Science Photo Library
Places of origin
Like Sutherland, Frances Westall, from the Centre for Molecular Biophysics in Orléans, France, sees the three key factors for life as having emerged by luck at the same time. Her view is that the reactions responsible would have happened on the surfaces of minerals. Chemists in her group talk about a ‘protocell’, with metabolic machinery and RNA contained in a tiny mineral pocket. Eventually, fatty lipid molecules would have formed an outer wall inside the pocket, giving cells the form we recognise today. “Depending upon the environment,” Westall says, “concentrations of lipids, proteins and RNA in porous mineral matrices would assemble to form a cell with a membrane.”
Westall and others are looking for the location where life emerged, studying mineral pores in deep-sea vents that spew out hot alkaline fluid. Her team has found possible examples in South African rocks (Westall et al., 2018). “Although temperatures at the vent may be greater than 300°C, far too hot to preserve proteins and other essential molecules, the external seawater is 2°C”, Westall says. The temperature gradient drives a flow of water that helps move compounds around, and may also help provide a driving force for metabolism. As Westall explains, “The vent edifices are full of reduced minerals that can be oxidised to provide energy for prebiotic reactions and surfaces for concentrating the organics, as well as various structural combinations and formations.” Other researchers have also suggested that, in such environments, cellular ion pumps – proteins that regulate the flow of ions across cell membranes – might have evolved (Lane & Martin, 2012).
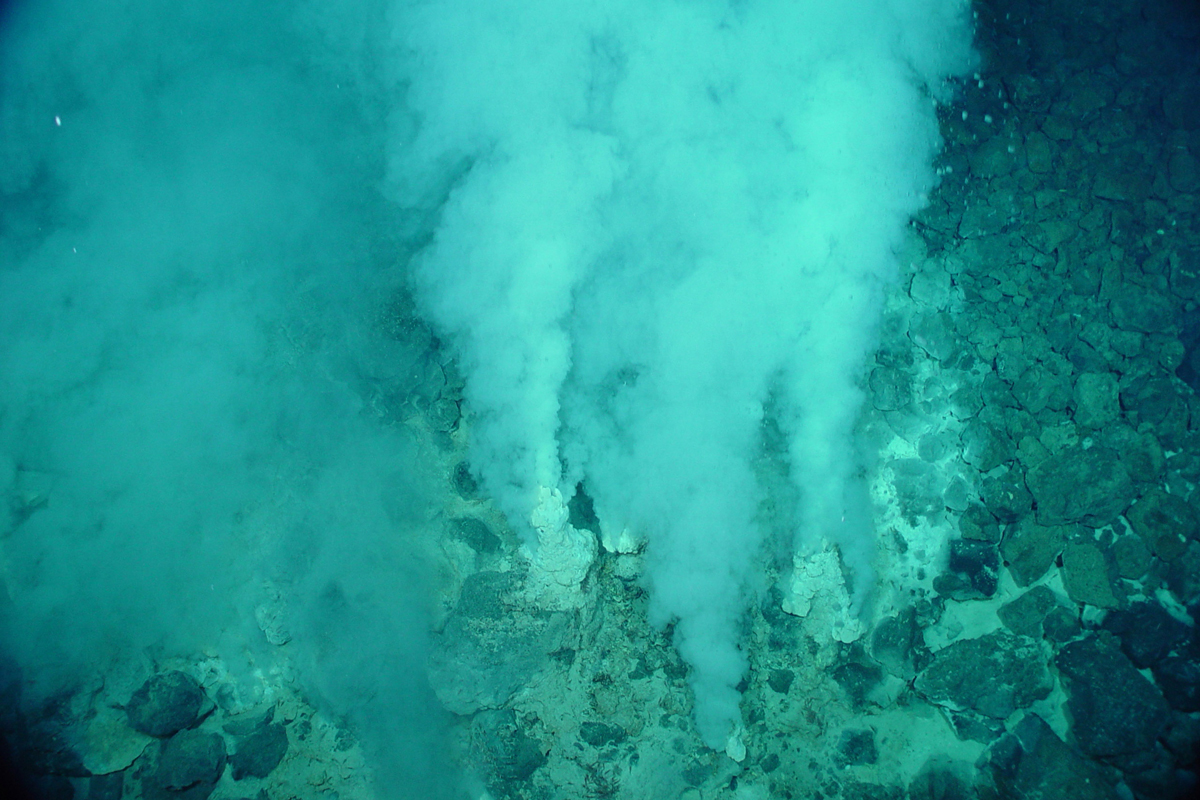
NOAA/Wikimedia Commons, public domain
Yet the search for places with the right chemistry and the energy to drive metabolism and enable cells to form also includes such locations as coastal volcanic areas, pumice rafts floating on the ocean, and geysers. A more speculative idea adds meteorites to this list: in 2014, rocks from the 24 km-wide Ries crater in southern Germany, which formed from a meteorite impact about 14.6 million years ago, were found to contain microscopic tubule-like formations, which have been linked to biological activity (Sapers et al., 2014). The tubules are similar to fossil traces of microbes found in volcanic glass, suggesting that water circulating within the rocks could have enabled microbes to colonise them. Some researchers have therefore suggested that similar tubules, formed billions of years earlier by meteorite impacts, could have offered a viable habitat for the earliest life forms.
Unusual science
Westall compares combining the different elements needed for the origin of life to developing cooking recipes. “If you want to get the perfect cake, you have to use exactly the right amount of specific ingredients and the right cooking temperature and time”, she says. “On the other hand, if you feel like experimenting, you change the ingredients, the quantities, temperature and time – and see what comes out.”
The idea of the three critical factors for life emerging all at once calls for something more like the second approach, Westall believes, and it’s an unusual kind of science. “Trying to do the experiments in a realistic scenario where you just stick in all the ingredients in a context that cannot be controlled, it just runs by itself, is really scary for chemists”, she says. “If something interesting happens, it’s more difficult to work out what and why. But that is the way to go now.”
References
- Lane N, Martin WF (2012) The origin of membrane bioenergetics. Cell 151(7): 1406-1416. doi: 10.1016/j.cell.2012.11.050
- Miller SL (1953) A production of amino acids under possible primitive earth conditions. Science 117(3046): 528-529. doi: 10.1126/science.117.3046.528
- Patel BH et al. (2015) Common origins of RNA, protein and lipid precursors in a cyanosulfidic protometabolism. Nature Chemistry 7(4): 301-307. doi: 10.1038/nchem.2202
- Powner MW, Gerland B, Sutherland JD (2009) Synthesis of activated pyrimidine ribonucleotides in prebiotically plausible conditions. Nature 459(7244): 239-242. doi: 10.1038/nature08013
- Ritson DJ, Sutherland JD (2013) Synthesis of aldehydic ribonucleotide and amino acid precursors by photoredox chemistry. Angewandte Chemie – International Edition 52(22): 5845-5847. doi: 10.1002/anie.201300321
- Sapers HM et al. (2014) Enigmatic tubular features in impact glass. Geology 42(6): 471-474. doi: 10.1130/G35293.1
- Westall F et al. (2018) A hydrothermal-sedimentary context for the origin of life. Astrobiology 18(3): 259-293. doi: 10.1089/ast.2017.1680
- Xu J et al. (2019) Prebiotic phosphorylation of 2-thiouridine provides either nucleotides or DNA building blocks via photoreduction. Nature Chemistry 11(5): 457-462. doi: 10.1038/s41557-019-0225-x
Resources
- Read more about scientific research on the origin of life in an article from Education in Chemistry.
- Listen to an episode of Sean Carroll’s ‘Mindscape’ podcast about how designing cells from scratch can help us understand how life arose.
- Read a feature from Current Biology to find out more about research relating to the origin of life.
Review
In almost all school textbooks, within the section that deals with the origins of life, references are limited to the famous experiment of Stanley Miller and Harold Urey in 1952. Since then, more experiments have been made, and it is certain that the question of the origin of life will always concern the scientific community.
This article, which explores several theories of the origin of life, can be used in a variety of ways – either as an example of the ways science evolves while basic research questions remain, or for the scientific theories related to living matter. It will be a journey through time, space and the world of great research questions.
Comprehension questions could include:
- When is life thought to have originated on Earth?
- What are the three factors that are believed to be fundamental for the emergence of life?
Panagiotis K Stasinakis, biology teacher and head of Ampelokipoi Laboratory Centre for Natural Sciences (EKFE), Greece