Titanic and the iron-eating bacteria Understand article
A species of bacterium discovered on the decaying wreck of the Titanic is providing new insights into how to protect living cells from damage.
The wreck of Titanic lies on the ocean floor, nearly 4 km below the surface of the dark, salty water of the North Atlantic. Divers on expeditions to the site have noticed ‘rusticles’ – icicle-like growths rich in rust – covering parts of the damaged and corroded hull. In 2010, scientists investigating rusticles taken from the Titanic site discovered a new species of bacteria within these growths w1. They named the new species Halomonas titanicae.
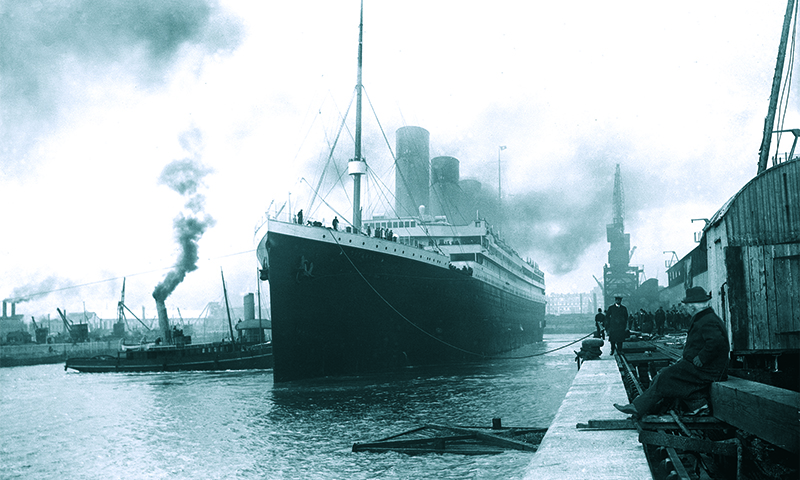
Wikimedia Commons (public domain)
The scientists have also discovered that Halomonas titanicae bacteria are involved in the rusting process and are accelerating the wreck’s decay. The decay is happening at quite a rapid rate: estimates suggest that the wreck may have disappeared completely by 2030. These bacteria could thus pose a significant threat to oil rigs and other iron structures located in the deep sea. However, these organisms have now become the focus of scientific research for another reason: to find out how they manage to thrive in conditions with high and varying salt concentrations.
Salts and the living cell
The term halo- means ‘salt’ in Greek. Bacteria of the genus Halomonas live in salty environments, such as sea water or salt marshes, where salt concentrations vary over a wide range. All members of the genus are strongly ‘halotolerant’: they have evolved ways of coping with wildly fluctuating salt concentrations outside the cell, surviving over a huge range of salinity from 0.5% (w/w) sodium chloride right up to 25%. This may seem like a trivial issue, but it is not.
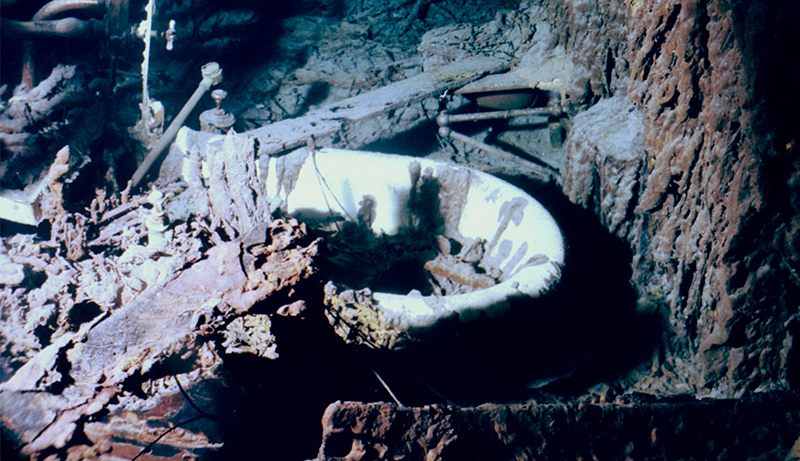
Image courtesy of Lori Johnston, RMS Titanic Expedition 2003, NOAA-OE
Cells are protected from the outside world by very thin membranes that control traffic in and out of the cell, creating and supporting huge differences in composition between the inside and outside of the cell. For example, cells maintain an electrical potential of about 100 millivolts across the membrane, which is typically just 10 nanometres thick. For all types of cell, sodium chloride (NaCl) is the dominant salt outside the cell (for example, in blood serum), whereas potassium chloride (KCl) is dominant inside the cell. Yet, while the salt types are different, the total salt concentration on either side of the cell membrane should match exactly. The reason for this is osmosis: if two solutions of different concentration are separated by a membrane that is permeable to water but not to solutes, water will flow from the more dilute side to the more concentrated side.
Cell membranes cannot cope with osmotic pressure differences. Red blood cells will burst when placed in pure water, illustrating the effect of osmotic pressure – and the fragility of cell membranes. Clearly, to keep their membranes intact, Halomonas bacteria must be able to regulate the concentration of solutes in the cytoplasm in response to changing external salt concentrations, without disrupting the biochemistry within the cell (see Zaccai 2009). How do they do this?
Halomonas bacteria produce large amounts of a substance called ectoine (see figure 1). To counterbalance the external salt concentrations, they adjust the concentration of this soluble compound within their cells. This keeps the cell’s fluids in osmotic balance with the outside, protecting the cell against shrivelling up or bursting even in extreme conditions. Remarkably, ectoine appears to act differently from most salts and solutes, which can interfere with the role of water in metabolic processes. Ectoine is a ‘compatible solute’, and so preserves the normal biochemistry within the cell. It also increases the stability of proteins and membranes. So how does this unusual solute achieve these results?
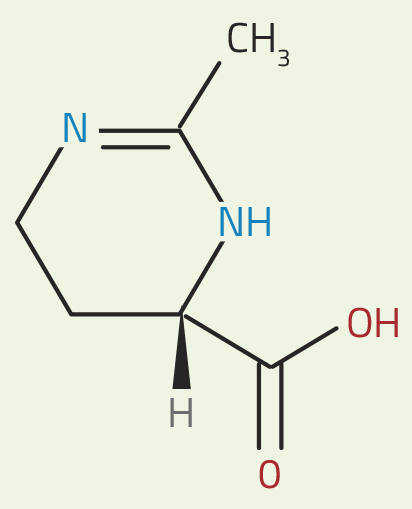
structure of ectoine. In water,
the red group becomes
negatively charged (by losing
one H+ ion) and the blue
group becomes positively
charged (by gaining one H+
ion). The charged groups
then form hydrogen bonds
with adjacent water
molecules.
Nicola Graf
Ectoine and the hydrogen-bond network
Clues as to exactly how ectoine works on the molecular level have recently been discovered using the advanced experimental technique of neutron scattering (see text box). Carried out by an international collaborationw2 involving the Institut Laue-Langevin (ILL)w3, the experiments showed how ectoine influences the layer of water around protein molecules and on membrane surfaces (Zaccai et al., 2016). Normally, water molecules interact with each other through a network of hydrogen bonds. The atoms in a water molecule interact with neighbouring molecules, with each oxygen atom acting as a receiver of two hydrogen bonds and each hydrogen atom as a donor of one bond. This results in a highly dynamic network of intramolecular bonding, with the molecules changing partners a billion (109) times a second.
The attraction between water molecules is also the basis of the ‘hydrophobic effect’, which makes oil and water separate. The hydrophobic effect is important in many biological processes, including the three-dimensional folding of protein molecules and the formation of membranes. Hydrogen bond dynamics are thus an essential factor in how living cells are organised.
Other substances in the water, such as salts, can interfere with this organisation. But, as the neutron experiments have revealed, ectoine seems to enhance hydrogen-bond dynamics – at least in part – rather than hindering it. This molecule contains a positively charged group (shown in blue in figure 1) and a negatively charged group (shown in red). These charged groups interact with water molecules, making the hydrogen bond network nearby a little less dynamic – but also creating a more dynamic network of water molecules further away.
Outside the bacterial context, ectoine is proving to be a useful ingredient in cosmetics and clinical treatments, because it can reduce inflammation in mammalian cells. The likely reason for this is that by stabilising proteins and membranes, ectoine protects human cells from damage, thus reducing inflammation and providing remedies for conditions such as allergies, eczema, and cough and cold symptoms.
Return to the Titanic
Although the neutron experiments have helped scientists to understand how Halomonas bacteria thrive in the potentially hostile environment around the sunken Titanic, the exact role of Halomonas titanicae in rust formation remains unclear. But there is some good news here: these iron-eating bacteria could have a role in future waste management, speeding up the decomposition of metallic litter – aside from historic wrecks – on the ocean floor.
Neutron scattering
Neutron scattering is a powerful method for studying the structure of materials, including water and its interactions with other compounds.
The nucleus of a hydrogen atom is a single proton. Neutrons and protons are very similar particles (apart from their electric charge), and neutrons strongly scatter off protons, rather like snooker balls bouncing off each other. The neutron scattering pattern provides information on the location of oxygen and hydrogen atoms in nearby water molecules, and thus on the dynamic network of hydrogen bonds that links them.
Another useful trick is used in neutron scattering. The nuclei of deuterium atoms (an isotope of hydrogen with one proton and one neutron in its nucleus) scatter neutrons in a very different way from normal hydrogen nuclei. Replacing the hydrogen in a specific compound (ectoine, for example) with deuterium makes it possible to see the contribution of only this compound to the scattering signal, distinct from that of the surrounding water molecules. This in turn helps to reveal the details of their interaction.
Neutron scattering with deuterium labelling has provided a rich crop of results in structural biology. These include insights into the complex interactions between protein molecules, and those between proteins and nucleic acids (DNA, RNA).
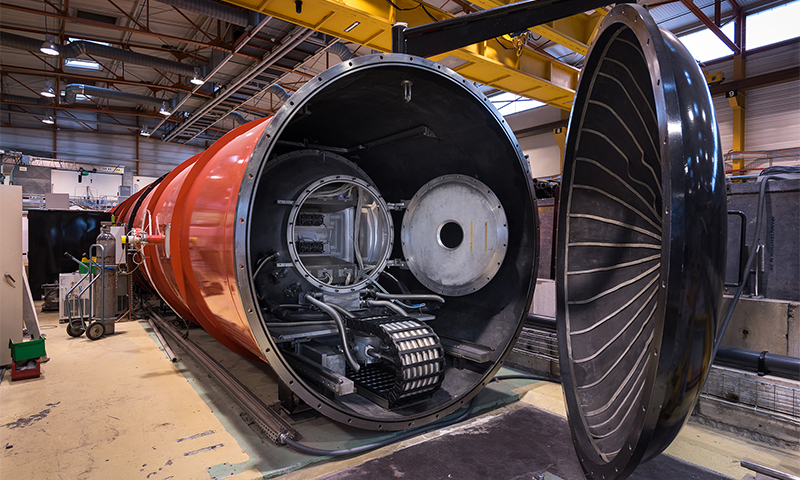
©ILL/L. Thion
References
- Zaccai G (2009) The intracellular environment: not so muddy waters. Science in School 13: 19-23.
- Zaccai G et al. (2016) Neutrons describe ectoine effects on water H-bonding and hydration around a soluble protein and a cell membrane. Scientific Reports 6: 31434. doi:10.1038/srep31434
Web References
- w1 – Halomonas titanicae bacteria were discovered by scientists from universities in Seville, Spain, and Toronto and Halifax, Canada. Read a scientific account of the discovery on the UNESCO website.
- w2 – The collaborating organisations included the Institut Laue-Langevin and Institut de Biologie Structurale in Grenoble, France, and the Max Planck Institute of Biochemistry and biotechnology company Bitop in Munich, Germany. See the online supplementary information section in Zaccai et al. (2016) for more information.
- w3 – The Institut Laue-Langevin (ILL) is an international research centre at the leading edge of neutron science and technology, based in Grenoble, France.
Resources
- Find out more about microbes that live on shipwrecks and the role of ectoine in an accessible article on the BBC website.
Institutions
Review
The wreck of the Titanic is very well known, so this article can be used to excite students about science. It can also act as an incentive to delve deeper into the world of bacteria and their usefulness to human beings – despite their normally negative associations.
The article would be useful in biology teaching to explore how prokaryotic organisms can survive in extreme environments, and how substances are transferred across the cell membrane. It could also be used in chemistry teaching about hydrogen bonds and the interactions between water and other molecules. Finally, recycling using Halomonas titanicae is relevant to ecology and the study of the energy balance within ecosystems.
Alina Giantsiou-Kyriakou, biology teacher, Livadia High School, Cyprus