Some (microbes) like it hot Understand article
Scalding volcanic springs are home to some remarkable microorganisms, and biotechnology is now finding uses for these microscopic survivors.
As a child visiting Yellowstone National Park, USA, in the 1990s, I remember being very excited to see the bright, paint-like colours in the hot springs. I was even more intrigued when I learned that the colours were due to microorganisms – living things that actually liked such extreme environments. I found it odd that anything could survive in conditions hot enough to give you a serious burn. Likewise, it amazed me when I later discovered that the dirt-digesting enzymes in washing powder come from organisms living at the opposite extreme of temperature – in water just a few degrees above freezing. Life, it seems, is content to thrive in conditions we once considered lethal. Several decades later, my love of chemistry and my fascination with these organisms has led to a career studying them in Iceland, which is now my adopted homeland.
What are thermophiles?
The diverse world of microbial life spans the whole temperature range, from below the freezing point of water to beyond the boiling point. Microbes that have a preference for hot environments are called thermophiles (Greek for ‘heat lovers’) and are just one of various kinds of ‘extremophile’ found living in extreme conditions. Thermophiles don’t merely tolerate heat – they positively thrive on it, growing best at temperatures above 50°C. Those with an optimum temperature range above 70°C are known as hyperthermophiles, and organisms that prefer cool environments (below 15°C) are called psychrophiles (see figure 1).
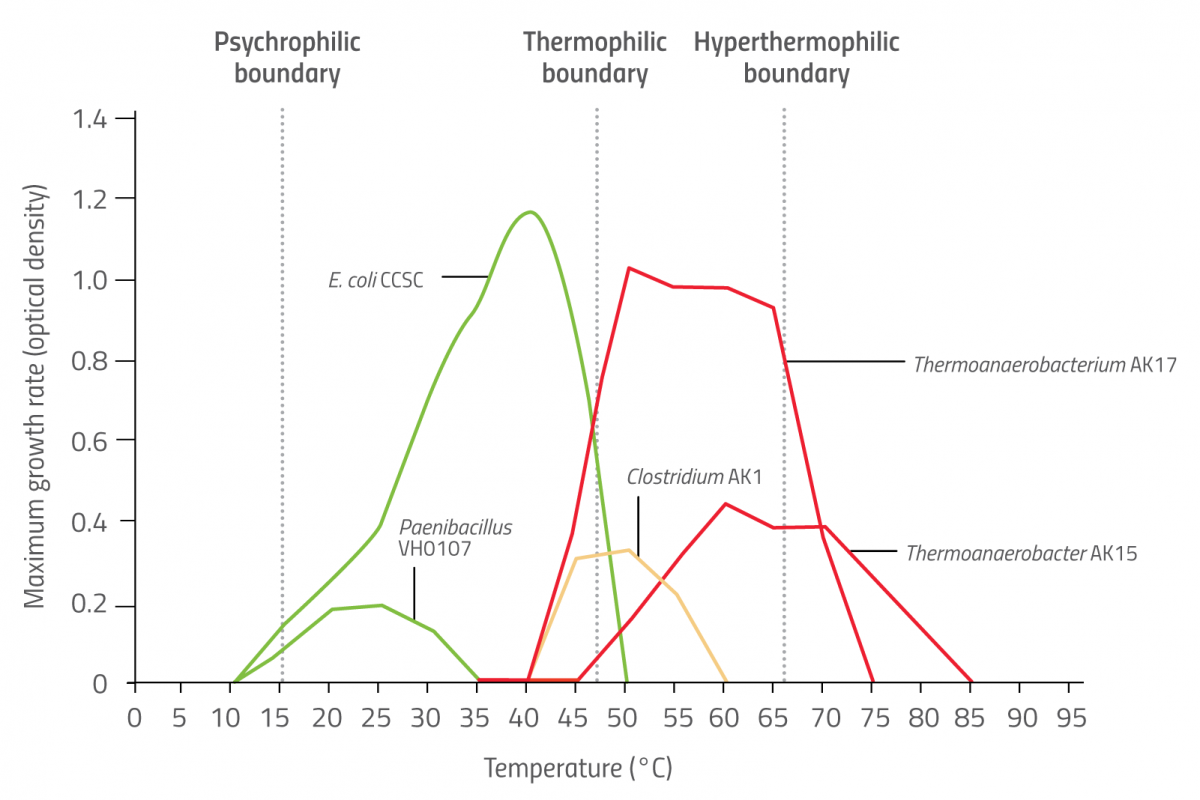
Sean Michael Scully/Nicola Graf
A few fungi and algae flourish in hot environments, but the vast majority of thermophiles are bacteria or equally tiny microorganisms known as archaea. These single-celled organisms were once classed as bacteria because they lack cell nuclei. Studies of their surprisingly complex DNA have revealed that archaea are actually more closely related to multicellular organisms such as humans than they are to bacteria. They are now considered to make up one of the three domains (superkingdoms) into which all forms of life are classified, alongside bacteria and eukaryotes (organisms with cell nuclei). Myriad types of archaea inhabit the scalding chemical soup found around volcanic vents on the sea floor. Some scientists think life began in such places, which suggests that these thermophiles have very ancient origins.
Here, there and everywhere
Since 1966, when thermophiles were first discovered growing in the hot volcanic springs of Yellowstone, they have been found in a huge range of places, from the deep sea to compost heaps, household water heaters, and the cooling pools in nuclear reactors. They even turn up in canned food: the bacterium Thermoanaerobacterium saccharolyticus can survive the sterilising process that destroys other microorganisms, and can then grow slowly at the lower temperatures inside the can, leading to a build-up of gas – sometimes with explosive results. The fact that thermophiles find their way into such diverse places suggests that their spores are widespread, waiting for the right conditions so they can spring back to life.
My particular interest is in the thermophiles that live around geothermal features such as hot springs, boiling mudpots, steam geysers, volcanic vents, and sulfurous steam vents called solfotaras. These sites are scattered far and wide across different parts of the globe, from Italy and Iceland to Yellowstone in the USA and Kamchatka in the Russian Far East. The geographical isolation of these island-like sites allows unique species to evolve in each, although some thermophile species can be found in many locations.
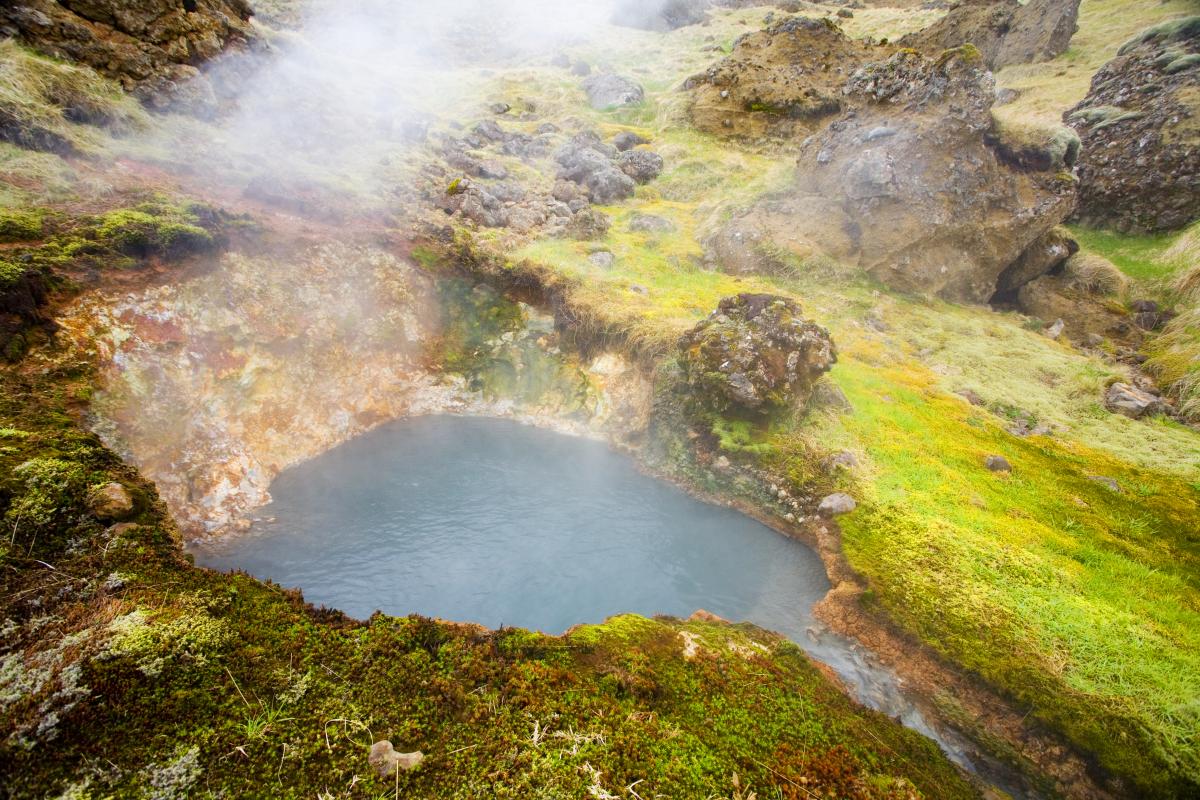
Sean Michael Scully
Another interesting feature of geothermal sites such as hot springs is that there is often a sharp temperature gradient: deeper underwater zones are hotter, while those towards the pool’s edge are cooler. Different thermophiles find a comfortable niche at different points along the gradient. Thermophiles that can photosynthesise, such as cyanobacteria, live at the cooler end of the gradient, while less brightly coloured types are restricted to the thermal extremes. The result is a multicoloured ‘microbial mat’ carpeting the rock, with each zone of colour representing the distinct biochemistry of the organisms present, from the yellow-green colours of those that photosynthesise to the diverse shades of those that obtain energy by metabolising hydrogen, iron or sulfur compounds. A famous example of colourful microbial mats is on display at Grand Prismatic Spring in Yellowstone, but the same phenomenon can be seen in geothermal features in Iceland and elsewhere.
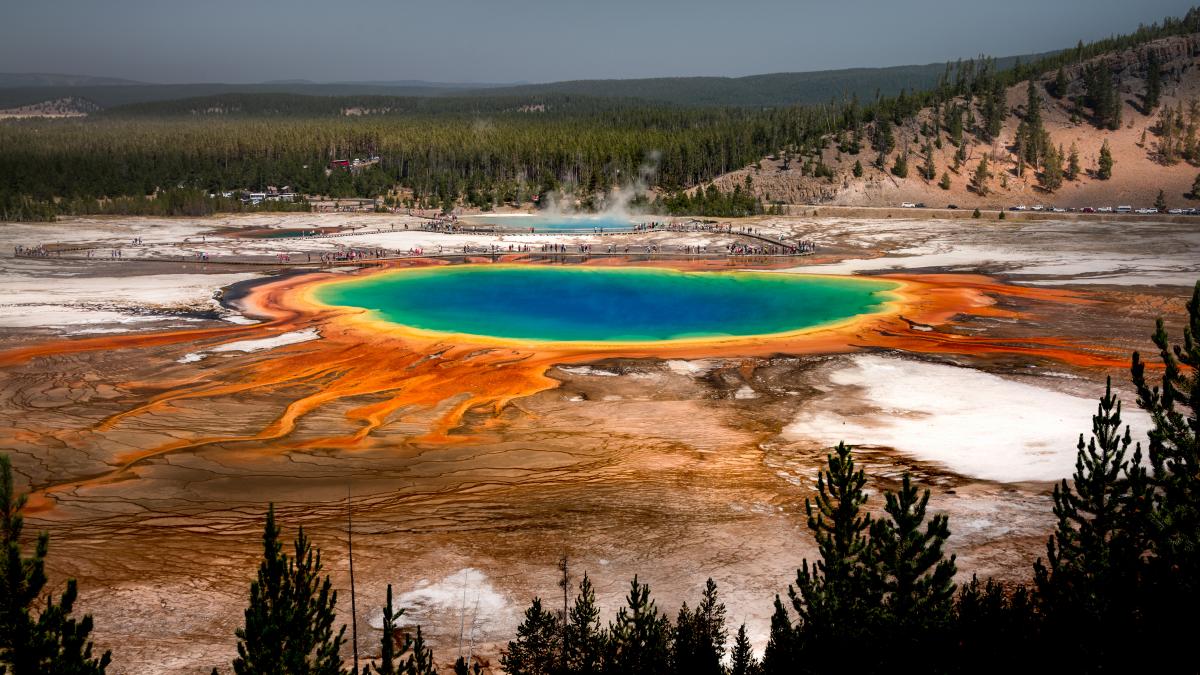
G_K_N/Flickr, CC BY-ND 2.0
Heatproof enzymes
Thermophiles owe their survival skills to their enzymes – the molecules that perform much of the chemistry of life, working as catalysts inside living cells. Enzymes are proteins, and most proteins are destroyed by heat. Fry an egg, for instance, and the heat denatures the protein molecules, making them unravel and change shape permanently. In contrast, thermophile enzymes are stable at high temperatures, allowing them not only to keep working but also to work faster than normal enzymes, since higher temperatures also mean faster reaction rates.
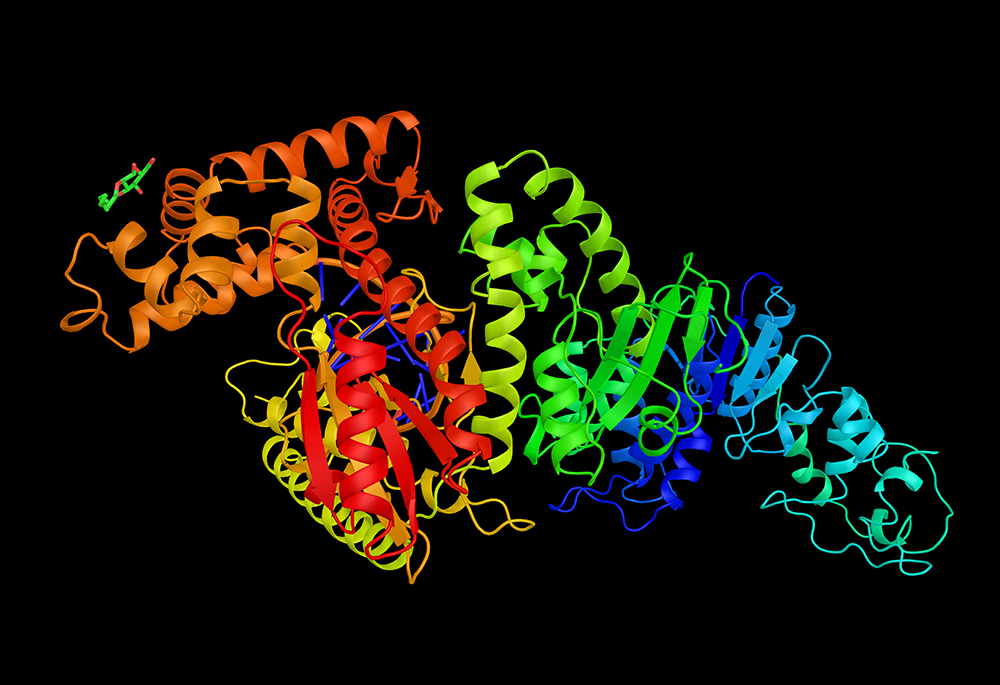
derived from a thermophilic
bacterium and used in DNA
replication
ibreakstock/Shutterstock.com
A famous example of a heat-stable enzyme is Taq polymerase, named after Thermus aquaticus, a thermophile found at Yellowstone. The Taq enzyme is now used in labs worldwide in a process called the polymerase chain reaction (PCR), which replicates DNA molecules from small samples. Before Taq, this was a laborious and expensive procedure that involved repeated cycles of heating and cooling, with fresh enzyme (a polymerase that denatured on heating) added at each cooling stage. Using Taq, the process is automated and runs at a high temperature, with only a small amount of the enzyme required. In a matter of hours, Taq-based PCR can turn a single DNA molecule into 100 billion copies, and a tiny sample of body fluid from a crime scene can thus yield enough genetic material to create a DNA fingerprint.
Although Taq PCR revolutionised molecular biology and forensics, thermophile enzymes are not just useful in genetics labs. They are also finding their way into a growing number of industries, from food and drink manufacture to fabric production. For instance, the high-fructose corn syrup used to sweeten soft drinks is made by heating a gloopy solution of corn starch to boiling point and digesting it with a succession of heat-tolerant thermophile enzymes. Keeping the solution hot has various benefits beyond speeding up the reaction: it makes the liquid less viscous and therefore easier to work with, and it keeps at bay the microorganisms that cause spoilage.
A boost for biofuels
Manufacturing sugary drinks more efficiently might not seem like a big step forward for society, but there are other applications of thermophiles that could prove to be of great benefit to the planet. Perhaps the most significant is the potential use of thermophiles to manufacture biofuels (Scully & Orlygsson, 2014).
Besides fossil fuels, the most abundant organic material on Earth is cellulose – the tough, fibrous carbohydrate made by plants to support their cell walls. Cellulose is all around us: it makes up most of the mass in wood, cotton, paper and all types of plant waste, from sawdust to crop stubble. If a procedure to convert cellulose efficiently into biofuel could be devised, it would provide a carbon-neutral alternative to fossil fuels.
Several thermophiles are capable of degrading cellulose to produce sugars or slightly larger carbohydrate molecules called oligosaccharides, consisting of several sugar molecules linked together. These products could then be fermented to produce alcohol or hydrogen, both of which make good fuels – and thermophiles can help with this second step, too. Although yeast is already widely used to produce alcohol from sugar, thermophiles can ferment a greater variety of sugars. The bacterium Thermoanaerobacter ethanolicus, for example, can digest many of the sugars that make up cellulose, such as xylose, galactose and mannose, producing alcohol (ethanol) as a product.
The bacteria Clostridium thermocellum and Caldicellulosiruptor saccharolyticus can even carry out both these steps themselves, breaking down cellulose directly and fermenting the resulting glucose to give a mix of products including hydrogen, acetic acid and ethanol.
Such species are the focus of ongoing research into biofuels, but there are hurdles to overcome. One challenge is to find a thermophile that can tolerate high levels of ethanol without being poisoned, a step that might require genetic modification. Alternatively, ethanol could be constantly removed from a continuous culture, preventing it from reaching toxic levels.
Future prospects
The technological potential of thermophilic organisms is tremendous, and so is their commercial potential. In 1991, the US biotech company that developed PCR from the Taq enzyme sold its patent for $300 million, but none of the proceeds found their way back to Yellowstone National Park, the original source of the enzyme. Today, strict guidelines govern what ‘bioprospectors’ can take from the site and ensure that any future profits derived from this genetic resource are shared. Such measures may help ensure that these unique and beautiful ecosystems survive to find uses in future biotechnology – and to inspire future generations of budding scientists.
References
- Scully S, Orlygsson J (2014). Recent advances in second generation ethanol production by thermophilic bacteria. Energies 8(1): 1-30. doi: 10.3390/en8010001
Resources
- Take a look at the author’s Facebook page about thermophilic anaerobes.
- Read about ‘hot bugs’ and how thermophilic bacteria can be used to produce hydrogen for fuel. See:
- Willquist K (2012) Hydrogen: the green energy carrier of the future? Science in School 22: 12-16.
Review
This article challenges readers to find out more about the microscopic living organisms called thermophiles and their amazing technological potential. Thermophiles are the exception to the rule in relation to their enzyme activity, so the article can be used as a stimulus for such topics as enzyme function, cell metabolism, and microbiology and its applications.
The article could also be used for a comprehension exercise, including questions such as:
- How does a cell’s physical environment affect its enzyme activity?
- How can we use thermophilic bacteria to protect our planet from the greenhouse effect?
It could also form the basis of an art lesson, with photos of colourful thermophiles acting as an unusual stimulus for student portrayals of nature’s beauty.
Alina Giantsiou-Kyriakou, biology teacher, Livadia High School, Larnaca, Cyprus