A particle accelerator in your salad bowl Teach article
Create a particle accelerator using a Van de Graaff generator, a ping-pong ball and a salad bowl to understand how it is used to study matter at the smallest scale.
Scientists are always on the hunt to find the smallest building blocks in the Universe. The atom, once believed to be indivisible, was the smallest fundamental particle until it was split to reveal electrons, protons and neutrons. More powerful particle accelerators revealed that protons and neutrons could be divided even further: each contains three quarks. The latest of these discoveries is a particle called the Higgs boson, observed in 2012 by the world’s largest particle accelerator – the Large Hadron Collider (LHC) at CERN.
Particle accelerators, which accelerate charged particles (such as protons or electrons) close to the speed of light, are used to study matter on the smallest scale. This enables scientists to better understand the properties of elementary particles, see how they interact and ultimately fathom how the Universe works. The theory that best describes particles and their interactions (all except gravity) is known as the Standard Model of particle physics. Since the model was finalised in the 1970s, it has successfully explained countless experimental results.
The quest is not over, though. It is known that the Standard Model describes only 4% of the Universe. Therefore, more experiments and more powerful tools are needed to explain the remaining 96%, including the enigmatic ‘dark matter’. The accelerators that are used to perform these experiments are huge, but you can show their basic operation in your classroom.
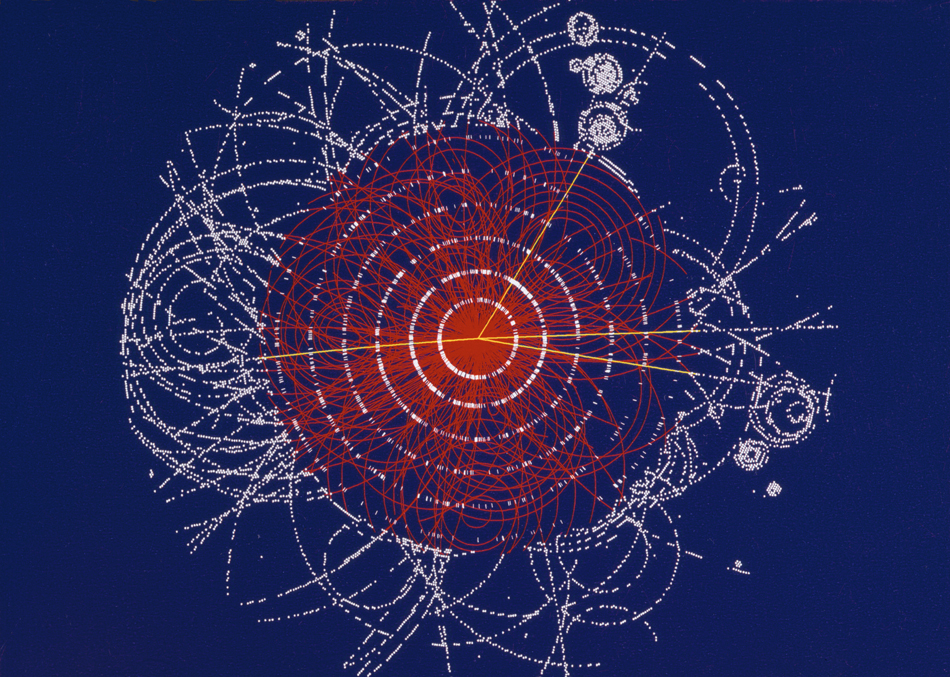
ATLAS / CERN
Creating a salad bowl accelerator
You may have experienced a shock from static electricity caused by friction, or perhaps seen how a Van de Graaff generator can make your hair stand on end. This device is used to charge a large metal sphere to high voltage. It was invented to supply the high energy required to propel charged particles in early particle accelerators, and the same principle is still used in electrostatic particle accelerators today.
Most modern large-scale accelerators, however, use changing electromagnetic fields: electric fields accelerate the particles to incredibly high speeds and magnetic fields control the beam of particles and its trajectory. This process can be demonstrated using a small Van de Graaff generator, a metal-coated ping-pong ball and a salad bowl. Students can construct the accelerator themselves, or the teacher can prepare it in advance of the lesson. A video outlining how to build a salad bowl accelerator (following the method below), and a paper cup accelerator, is available on YouTubew1.
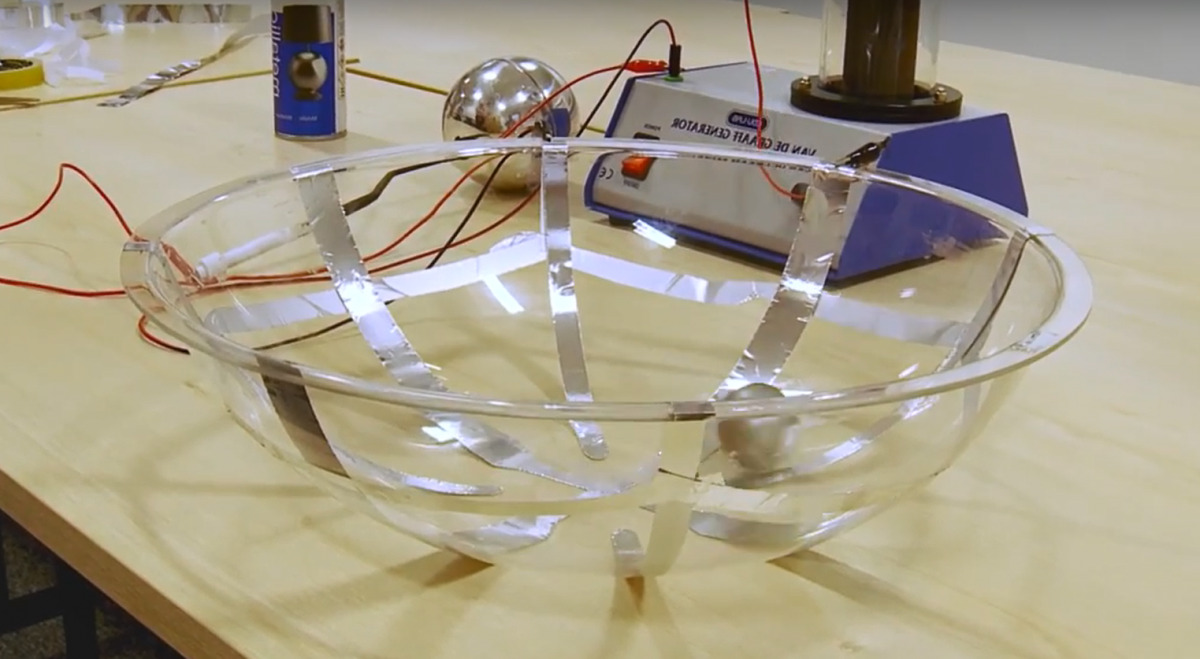
Cockcroft Institute
Materials
- Plastic salad bowl (not glass): it should be smooth and with a shallow curve – any bump or dent will hinder the ball’s trajectory
- One ping-pong or polystyrene ball
- Conductive paint: either nickel or graphite (to create the electrically conductive coating)
- Paint brush
- Aluminium (or copper) foil adhesive, at least 2 cm in width
- Van de Graaff generator (or Wimshurst generator)
- Electrical wires, banana plugs, crocodile clips
- Scissors
- Toothpicks (to hold the balls while painting and drying them)
- Newspapers (to protect surfaces when painting)
- Plastic spoons (to manipulate the balls when in the bowl)
Safety note
The voltage generated in a Van de Graaff can be very high (several tens of thousands of volts) but the current is low. That means that the electric shock is not dangerous to a healthy person, but it’s still painful and should be avoided. Operation of the Van de Graaff generator should be supervised by a trained adult (the teacher or demonstrator) and the following rules must be followed:
- Avoid touching or getting too close to the charged surfaces.
- Make sure that the earth clips are firmly attached.
- Always discharge the dome after use by touching it with an earthed object.
Method
- Prepare the ping-pong ball by coating it in conductive paint. As the paint will need some time to dry, you may want to start painting the ball a few days before preparing the salad bowl.
- Cut two pieces of aluminium tape. Each should be about 2 cm wide and long enough to go from one rim of the bowl, along the bottom of the bowl and up to the other rim.
- In the middle of these strips, trim each side so they are narrower (about 1 cm in the centre) than the rest of the strip, as in figure 1.
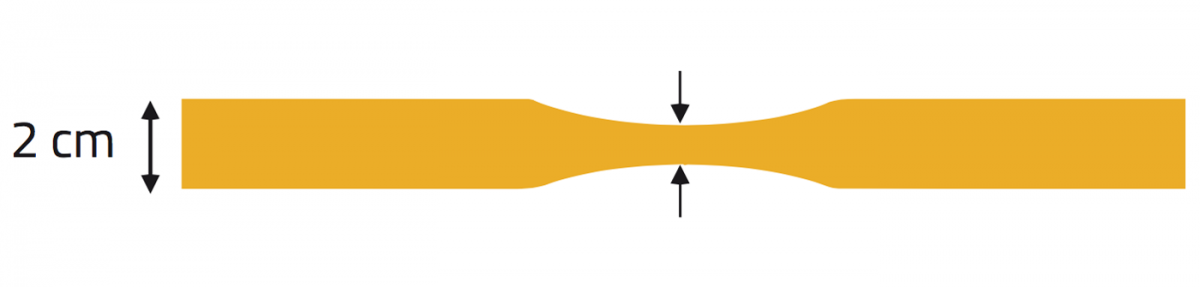
Cockcroft Institute
- Stick the strips onto the bowl: they should be at a 90° angle, forming a cross at the centre of the bowl. The strips should end at the edge of the bowl (figure 2).
- Cut eight short, narrow strips (about 1 cm wide) from another piece of aluminium tape. They should be long enough for one end of the strip to run over the edge of the bowl when the other end is placed close to the centre of the bowl. Round the ends of the strips as charge may leak from sharp corners. Note that the wider strips in step 3 do not need to be rounded.
- Using four of the narrow strips, stick one strip in each of the spaces between the cross. They should not be touching the centre. The end of the strips should run over the edge of the bowl and down the outside (figure 3).
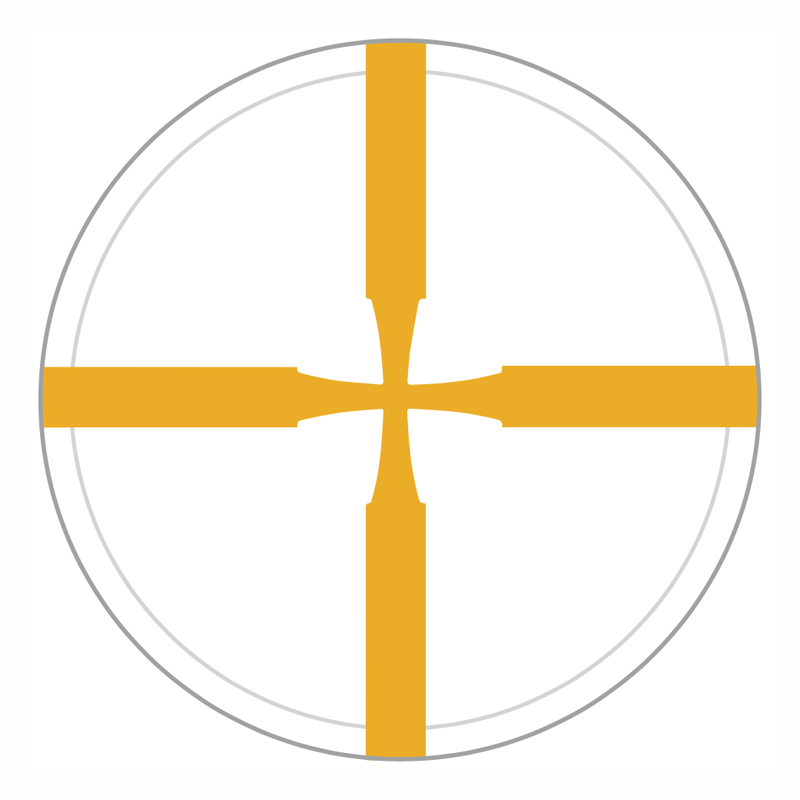
Cockcroft Institute
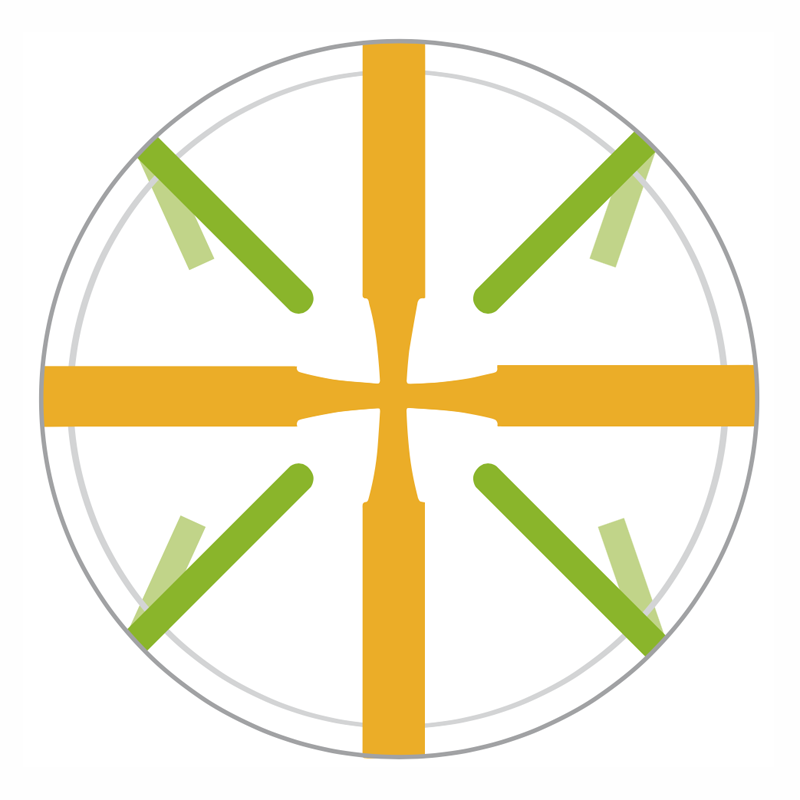
Cockcroft Institute
- With the remaining four narrow strips of tape, join all the narrow strips together on the outside of the bowl (figure 4).
- Use aluminium tape, crocodile clips and banana plugs to connect one of the narrow strips at the edge of the bowl to the earth terminal of the Van de Graaff generator and connect the cross to the high-voltage terminal (the dome) of the Van de Graaff generator (figure 5). Make sure the high-voltage and earth leads do not touch each other.
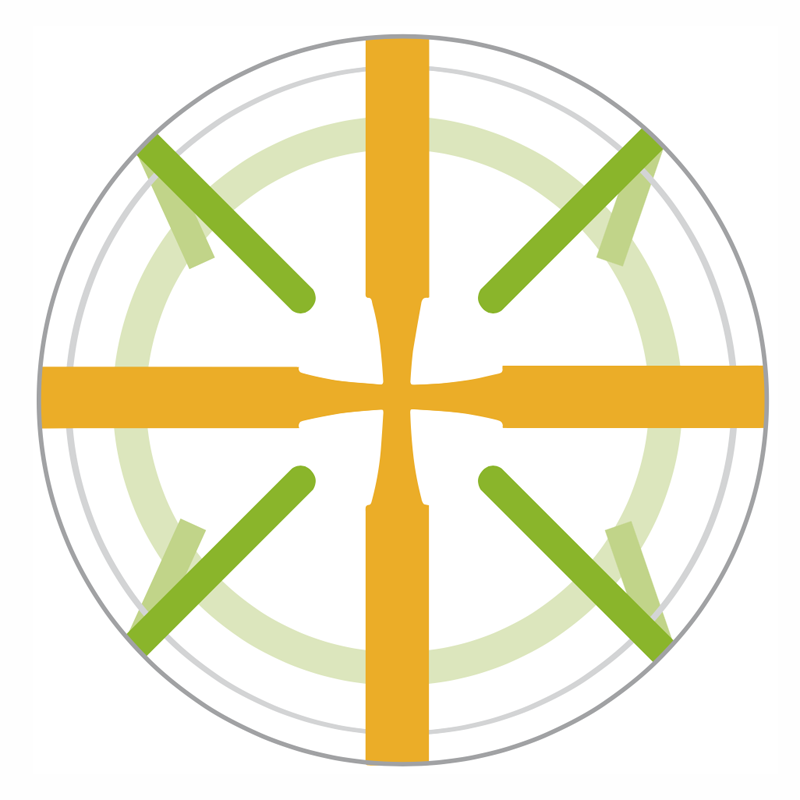
Cockcroft Institute
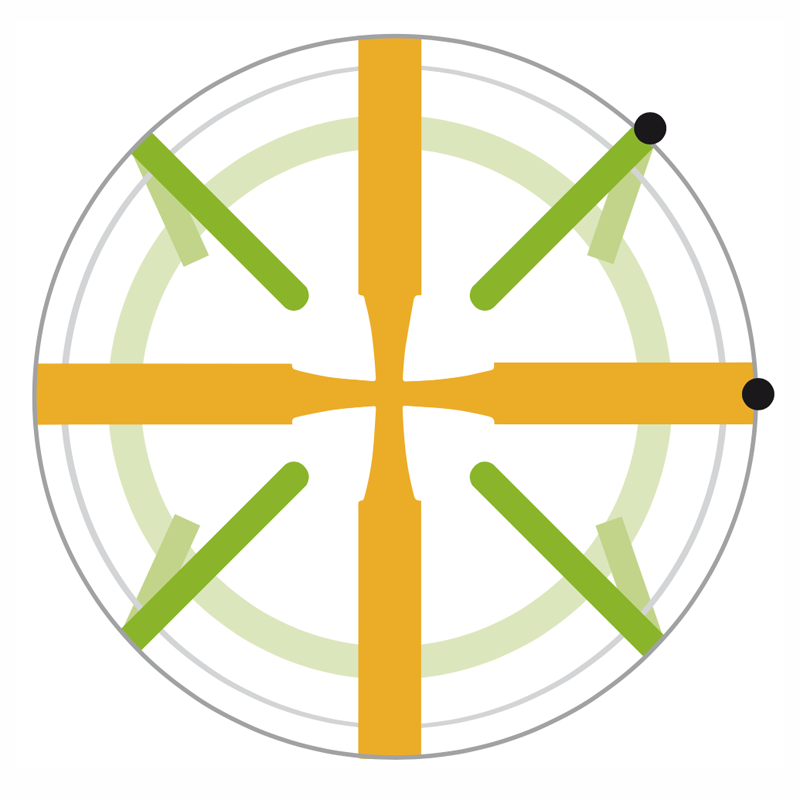
Cockcroft Institute
- Drop the ball into the bowl and switch the generator on.
- Watch the ball spin inside the bowl. After the experiment, don’t forget to discharge the Van de Graaff generator.
Troubleshooting
If the ball does not begin to spin:
- Check that the generator is on
- Nudge the bowl gently to initiate motion
- Check the live strips and wires are not touching the earthed ones
- Try using a smaller ball.
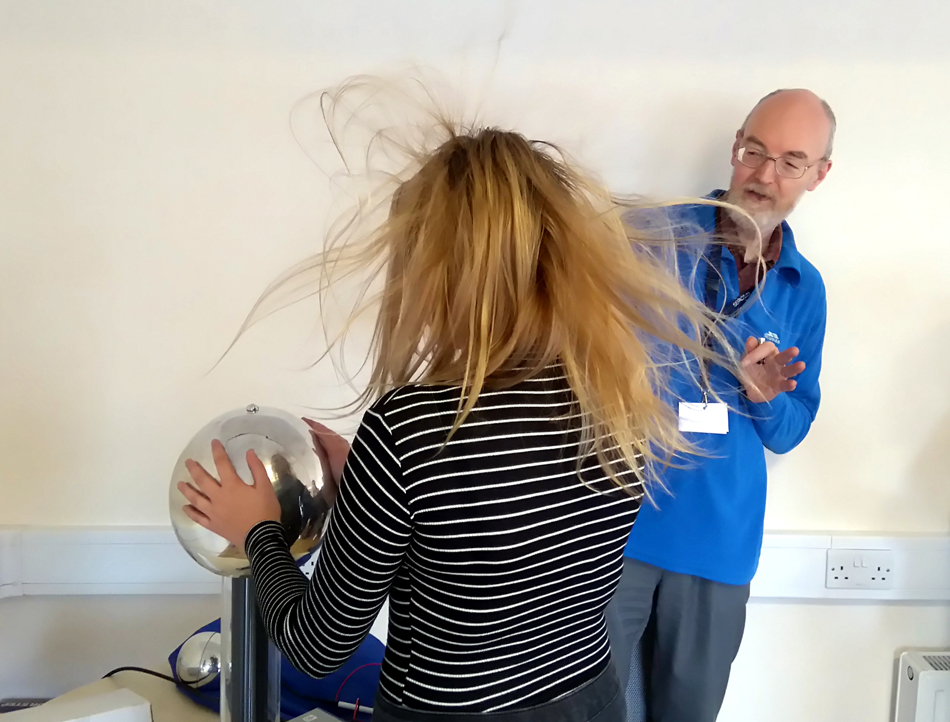
Cockcroft Institute
How does it work?
The Van de Graaff generator produces static electricity, which builds up a high voltage (over 30 000 volts) on the metal strips forming the cross. This means that these metal strips are charged (either positively or negatively). However, the current that is actually flowing is very small, which makes the whole procedure safe. The other strips are earthed so they hold no charge.
The moment the ball comes into contact with one of the charged strips, it picks up that charge. Because both the ball and the strip now have the same charge, they repel each other: the ball moves away. It then rolls onto an earthed strip, causing the ball to neutralise and lose its charge. The ball is accelerated every time it touches a charged strip and decelerates in between strips due to the friction with the bowl.
How is it similar (and different) to a real accelerator?
The salad bowl accelerator is a simple model for explaining the workings of real particle accelerators, such as the LHC. Although the same principle of the original Van de Graaff accelerator is still used today, modern accelerators have some fundamental differences.
Particle charge and electric fields
Unlike the particles in an accelerator, the ball in the salad bowl has no charge of its own – its charge changes depending on the aluminium strip that it was last in contact with. In CERN’s LHC, the protons and ions each have their own charge, which does not change. Instead, specially designed metallic chambers are spaced around the accelerator, which oscillate between negative and positive charge, creating radio waves that push the particles forward in bunches.
In this activity, the ball represents one of the particles that scientists want to accelerate and collide. However, in a real collider, there are billions or even trillions of these, which together form a beam. To avoid collisions with air molecules, the beam travels in a vacuum inside a metal pipe. Monitoring the properties of this beam in real time is extremely important, for example to protect the machine, so scientists use a variety of techniques called beam diagnostics to track every single particle in the beam.
Size and shape
Particle accelerators can be linear, whereby the beam of particles travels in a straight line from one end to the other, or circular, in which the beam of particles travels around in a loop. The advantage of a circular accelerator, such as the LHC, is that with each loop, the particles accelerate a little bit more. This makes it possible to reach high energies, although the particles do lose some energy in each turn due to an effect called synchrotron radiation. The final energy of a circular accelerator is limited by its size, the intensity of the accelerating fields and of the bending magnets which keep the particles on track. A typical salad bowl is about 30–40 cm wide, whereas the LHC has a diameter of 8.5 km. The LHC’s huge size is one of the reasons why it can accelerate particles almost to the speed of light.
In the salad bowl accelerator, the speed of the ball is limited by friction and by the rate of charge transfer between the strip and the ball. When the ball moves slowly, it remains on the strips for longer and has more time to charge and discharge, so there is more scope for acceleration. When the ball moves faster, it spends less time on the strips and eventually reaches a constant final velocity.
Magnets
Magnets are essential for controlling the unruly particles that circulate within both linear and circular accelerators. For example, quadrupole and higher-order magnets are used to squeeze the particles together and to control the size and the divergence (how much the diameter of the beam increases with distance) of the beam.
In circular accelerators, the particles also need to travel in a curve rather than a straight line, which is achieved using very strong dipole magnets. The higher the energy of the particle beams, the stronger the magnets need to be. The LHC’s main magnets are superconducting, meaning they conduct electric current with zero resistance when they are cooled to sufficiently low temperatures. These magnets operate at a temperature of 1.9 Kelvin (–271 °C), which is achieved by pumping 70 000 litres of liquid helium through a closed circuit around the accelerator. The salad bowl itself works like the magnets, constraining the movement of the ball and forcing it to travel in a circle – luckily, this works at room temperature.
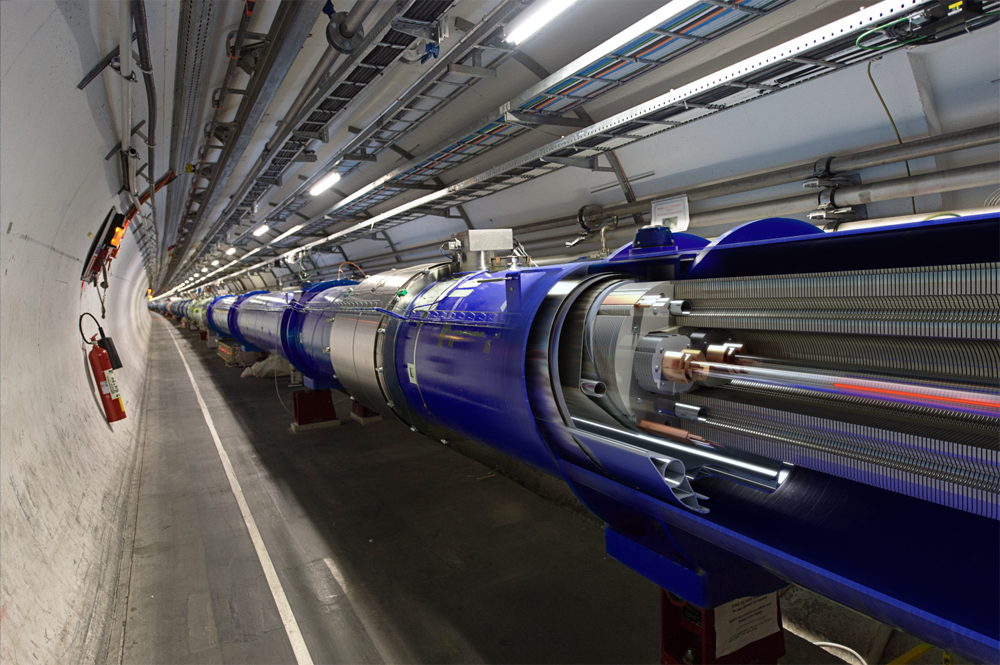
CERN / Daniel Dominguez
Particle detectors
Accelerating particles is only the first half of the story. Particle accelerators increase the speed of particles to high energies before they are directed at a fixed target (such as a thin piece of metal foil) or made to collide by aiming two beams of particles at each other. In accelerators such as the LHC, two particle beams travel in opposite directions, one clockwise and the other anticlockwise, and they are made to collide almost head-on at specific points. Detectors are placed around the target point to gather information about the particles that result from the collision, such as their speed, mass and charge. From this information, scientists may discover new particles or find out how the particles interact with each other. This was how researchers at CERN discovered the Higgs boson, a particle believed to give mass to all the other particles in the Universe.
Questions for the students
Why is the ball spinning in a particular direction?
Whether the ball spins in a clockwise or anticlockwise direction is determined by the initial impulse given to the ball. If the ball starts at rest, it will move away from the charged stripe in one direction or the other depending on the exact position of the ball, so it is difficult to predict. After a few random movements, a general direction sets in and the ball starts to accelerate.
Could you make the ball spin the other way around? How?
Just take the plastic spoon and give it a push in the opposite direction.
How could you make the ball spin faster?
The speed of the ball is limited by friction and by the rate of charge transfer between the strip and the ball. Having a smoother ball and bowl would allow the ball to reach final velocity more quickly, but it would not change the final velocity itself. The shape of the bowl is important because the final trajectory of the ball is determined by the balance between gravity, the centrifugal force and the normal force. The normal force prevents the ball falling through the plastic bowl, and because the force is perpendicular to its surface, it depends on the shape of the bowl.
What happens if you use an uncoated ball? Why?
Without the conductive coating, the ball could neither charge nor discharge, so it would not accelerate.
CERN / Maximilien Brice
Next generation colliders
Particle accelerators are complex and cutting-edge machines whose development usually requires collaborations between people from different countries, working in very different fields. It took almost 30 years for thousands of scientists, engineers and technicians to plan and build the LHC.
While the world’s largest particle accelerator still has several decades left of activity, researchers from institutions all over the world are already putting their heads together to design the next generation of colliders. The replacement for the LHC will probably enter service in the 2040s, so most of the scientists who will operate it are currently in school. The salad bowl accelerator may just give them a head start.
Acknowledgement
The inspiration for this activity came from physicists Todd Johnson (Fermilab) and Suzie Sheehy (Oxford University) who work in the field of particle acceleration.
Web References
- w1 – Watch a video from the Cockcroft Institute showing how to build salad bowl and paper cup accelerators.
- w2 – Connect with the University of Liverpool Physics Department and discover their outreach opportunities.
- w3 – The Cockcroft Institute is an international centre for accelerator science and technology in the UK. It is a joint venture between the universities of Lancaster, Liverpool and Manchester and the Science and Technology Facilities Council (STFC) at the Daresbury and Rutherford Appleton Laboratories.
Resources
- Explore how accelerators work and what their main components are using AcceleratAR, an augmented reality app.
- Discover what accelerators are used for and why they are important by watching this series of talks from the University of Liverpool.
- Visit the CERN website to learn more about the LHC.
- Watch a webinar from the Institute of Physics on the top five static electricity demos, including the salad bowl accelerator.
- Further information and alternative instructions for the salad bowl accelerator activity are available from the Science & Technology Facilities Council and the International Particle Physics Outreach Group.
- To learn how to build your own Van de Graaff generator and linear accelerator, watch these videos on YouTube from the Cockcroft Institute:
- Read more about the LHC, the building blocks of matter and the standard model.
- Landau R, Rau M (2008) The LHC: a step closer to the Big Bang. Science in School 10: 26–33.
- Learn more about how the LHC works and read about some of the experiments being carried out at CERN.
- Landua R (2008) The LHC: a look inside. Science in School 10: 34–45.
- Read the story behind the Higgs boson.
- Hayes E (2012) Accelerating the pace of science: interview with CERN’s Rolf Heuer. Science in School 25: 6–12.
- Create a particle accelerator using a cathode ray tube to explore the principles of the LHC.
- Brown A, Merkert J, Wilson R (2014) Build your own particle accelerator. Science in School 30: 21–26.
- A physics teacher explains how his time as a teacher in residence at CERN has inspired both him and his students.
- Watt S (2012) Nuclear options: a teacher at CERN. Science in School 23: 57–60.
- Bring particle physics to life in the classroom with the aid of a homemade particle detector.
- Barradas-Solas F, Alameda-Meléndez P (2010) Bringing particle physics to life: build your own cloud chamber. Science in School 14: 36–40.
Review
This article models the operation of a synchrotron particle accelerator. It’s great fun to build and even more exciting to operate and watch. A brief history of the use and operation of particle accelerators is included. This would be the perfect way to prepare for a trip to Diamond Light Source in the UK or CERN in Switzerland.
Comprehension questions could include:
- What are fundamental particles?
- Why are particle accelerators important to scientific research?
- Can the cost of particle accelerators be justified?
- Why can the building and operation of a particle accelerator be classed as multidisciplinary?
Robert Woodman, Head of Science, Ysgol Bro Gwaun, UK