Fighting HIV with neutrons Understand article
When thinking about diffraction studies, X-rays most often come to mind, but neutrons can also provide important structural information – and could help in the fight against HIV.
When you think about crystallography, the technique that most often comes to mind is X-ray diffraction. It’s no wonder: in biology, more than 88 000 structures of proteins, nucleic acids, viruses and macromolecular assemblies have been determined using X-rays. But as neutron crystallography has improved, it is becoming increasingly useful in helping to design drugs. Researchers at the Institut Laue-Langevin (ILL) in Grenoble, France, have recently used the facility to understand how an anti-retroviral drug targets HIV.
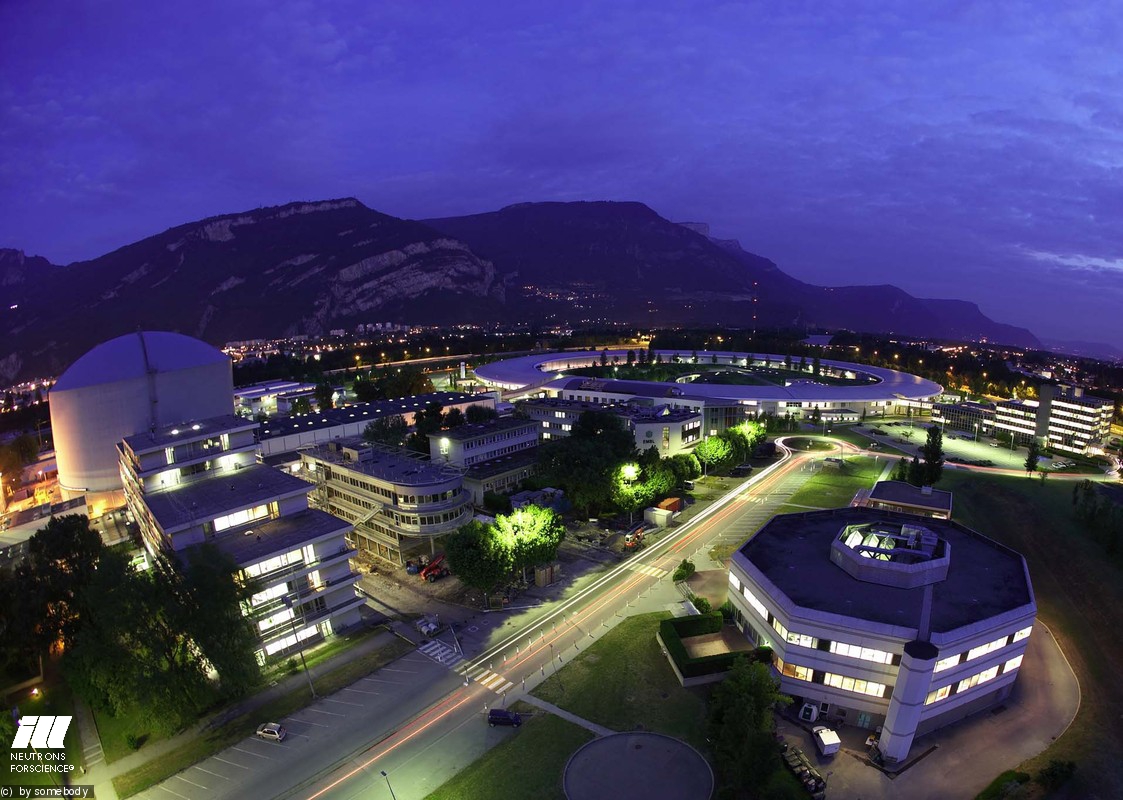
research neutrons in the world.
Image courtesy of ILL
Since the early days of protein X-ray crystallography around one hundred years ago, the technique has undergone dramatic developments and become widespread. High-intensity X-ray beams provided by synchrotron radiation sources allow data to be routinely collected from tiny crystals just micrometres across and in a matter of seconds (see Cornuejols, 2009).
In contrast, the development of neutron crystallography of large biological molecules has been far less pronounced and its application much less widespread. The main reason for this is that the number of particles per square centimetre per second (known as fluxes) from even the most intense neutron sources are many orders of magnitude less than the corresponding fluxes at X-ray sources.
That meant that until recently, neutron crystallographers needed large crystals and exposure times of months to collect sufficient data. Recent improvements, however, allow enough data to be collected from much smaller crystals in just a few days. That’s still longer than many X-ray-based experiments though, so why would we use neutrons? One of the reasons is the smallest atom in the Universe: hydrogen.
The importance of hydrogen
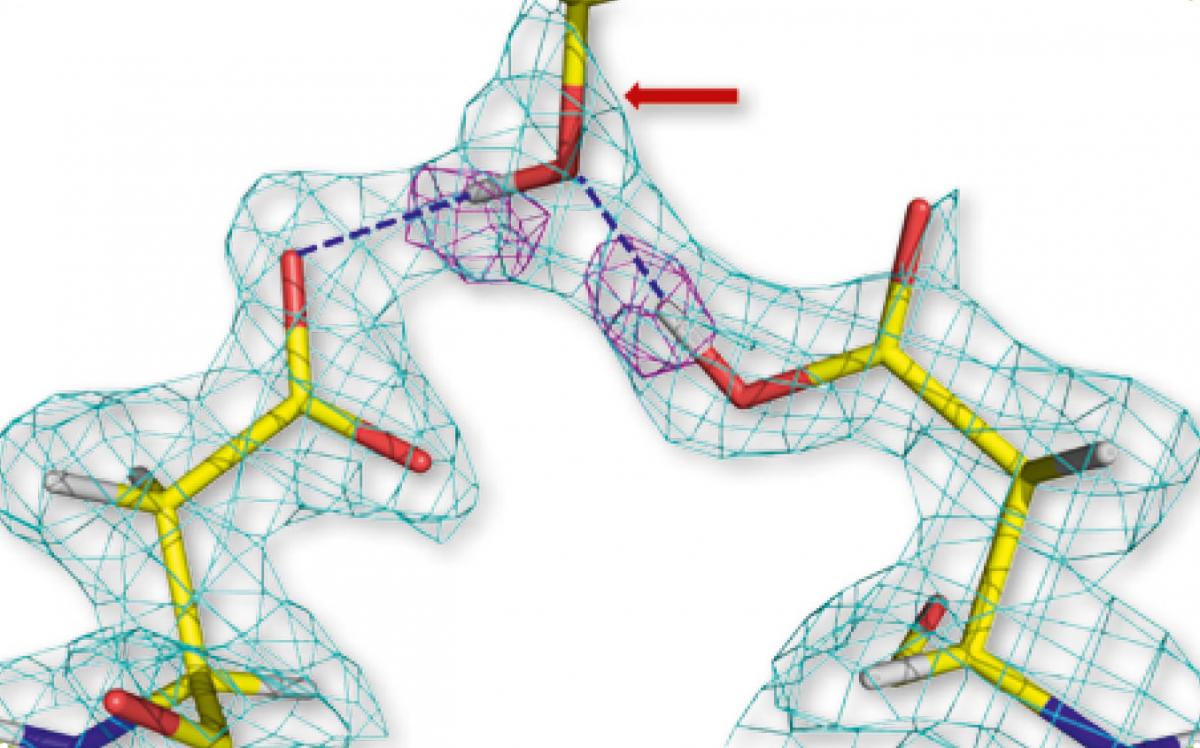
residues of two HIV-1
protease molecules (left and
right), hydrogen-bonded to
the hydroxyl group of the
drug aprenavir (centre). The
pink geometric shapes
represent hydrogen atoms
Image courtesy of Andrey
Kovalevsky
Most drugs work by binding to a specific enzyme involved in a disease, so that its function is inhibited. A lot of modern drug design therefore focuses on analysing and optimising the interactions between the drug and its target. X-ray crystallography has been the favoured method to unravel these structural details, but X-rays do not generally reveal the position of hydrogen atoms in a molecule. These often play a crucial role in binding through weak yet important interactions known as hydrogen bonds.
In contrast, neutrons can locate the positions of all atoms including hydrogen, and so they provide a powerful analytical tool for analysing drug-binding interactions. Recently, this was demonstrated in a study of the binding between an anti-retroviral HIV drug (amprenavir) and its target enzyme, HIV-1 protease. This enzyme is a key part of the HIV life cycle – it breaks down viral polypeptides to create the proteins needed for the maturation and the production of new infectious virus particles.
Scientists fired neutrons at a tiny crystal of HIV-1 protease bound to amprenavir (only 0.2 mm3 in size) to collect data at a resolution of just 0.2 nanometres. These data allowed researchers to locate the hydrogen atoms in the enzyme–drug complex – and, critically, to identify those atoms participating in hydrogen bonding between the drug and the enzyme.
Better resolution
Using previous X-ray studies, scientists had speculated that several hydrogen-bond interactions were important in the binding of HIV-1 protease and amprenavir. However, the neutron study revealed that, in fact, only two strong, direct hydrogen bonds exist between the drug and the enzyme (figure 1). This finding shows drug designers new ways to strengthen this binding by subtly modifying the drug’s molecular structure. Hopefully these changes will increase the effectiveness of the drug and reduce the necessary dosage.
For example, drug designers could make the two hydrogen bonds even stronger by adding a reactive atom such as fluorine to the drug. Alternatively, they could add more direct hydrogen bonds, for example by incorporating larger groups of atoms into the structure that would expel water molecules currently found in the binding site.
The unique sensitivity of neutron analysis to hydrogen atoms provides the pharmaceutical industry with a new and powerful tool for structure-guided drug design. Although the value of X-ray macromolecular crystallography will certainly continue for many years because of its higher resolution, using both X-rays and neutrons reveals more clearly how drugs interact with their protein targets and will no doubt improve the efficacy of other pharmaceuticals in the future.
Neutron diffraction
When a crystal is placed in an X-ray beam, the X-rays interact with the electron clouds of the atoms in the crystal, causing them to diffract in specific directions. By measuring the angles and intensities of these diffraction spots – or ‘reflections’ – we can produce a three-dimensional picture of the electron density within the crystal, from which the mean positions of the atoms can be determined.
Neutron diffraction is conceptually very similar, except that the neutrons are diffracted by the atomic nuclei of the crystal lattice rather than the electron clouds around them. The scattering strengths for different atom types therefore don’t correlate with electron configuration but with the nuclear forces, which can even vary between different isotopes of the same element.
Because of their small electron clouds, hydrogen and deuterium don’t have much effect on X-rays but they have similar neutron scattering strengths to the other elements common in biological macromolecules: carbon, nitrogen, oxygen and sulfur. Consequently, hydrogen and deuterium, are not visible using X-ray scattering but are visible with neutron scattering.
This visibility of hydrogen and deuterium allows their positions to be determined at resolutions of around 1.5 and 2.5 Å (1.5 x 10-10 m and 2.5 x 10-10 m), respectively. A hydrogen atom is about 1 Å across. This makes neutron macromolecular crystallography particularly useful for studies in which a knowledge of hydrogen atom positions is important, such as studies of enzyme mechanisms or drug-binding studies where details of the hydrogen-bonding interactions can help guide drug design.
More about ILL
The Institut Laue-Langevin (ILL)w1 is an international research centre based in Grenoble, France. It has led the world in neutron-scattering science and technology for over 40 years, since experiments began in 1972. ILL operates one of the most intense neutron sources in the world, feeding beams of neutrons to a suite of 40 high-performance instruments that are constantly upgraded. Each year 1200 researchers from over 40 countries visit ILL to conduct research into condensed matter physics, chemistry, biology, nuclear physics and materials science.
ILL is a member of EIROforumw2, the publisher of Science in School. See the list of all ILL-related articles in Science in School.
References
- Cornuejols D (2009) Biological crystals: at the interface between physics, chemistry and biology. Science in School 11: 70–76.
Web References
- w1 – Get more information about ILL
- w2 – EIRO forum is a collaboration between eight of Europe’s largest inter- governmental scientific research organisations, which combine their resources, facilities and expertise to support European science in reaching its full potential. As part of its education and outreach activities, EIROforum publishes Science in School.
Resources
- Neutron diffraction has also helped researchers investigate how antifreeze in Arctic fish blood keeps them alive in sub-zero conditions. Read more at:
- Blakely M, Hayes E (2011) Neutrons and antifreeze: research into Arctic fish. Science in School 20: 18–22.
Institutions
Review
This is an interesting article which demonstrates how advances in different branches of science, in this case neutron diffraction, may be used in the development of new drugs for the benefit of mankind.
The article should serve as useful background reading for teachers and may be used to complement the teaching of science with real-life applications. It can also be used in comprehension or discussion activities especially with older students. The type of questions asked depend on what the teachers would like to teach or focus on. For example, what is the advantage of using neutrons instead of X-rays?
Paul Xuereb, Malta