Cold seeps: marine ecosystems based on hydrocarbons Understand article
David Fischer takes us on a trip to the bottom of the sea to learn about cold seeps – their ecosystems, potential fuels, and possible involvement in global warming.
What are cold seeps?
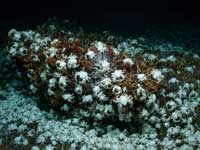
grazing on an extensive
mussel bed at a cold seep
off the coast of Pakistan
Image courtesy of MARUM,
Bremen University
Cold seeps are often oases for microbial and macrofaunal life on the sea floor – similar to hydrothermal vents, where hot water emerges under high pressure, several kilometres below the sea (see Little, 2010). In contrast to hydrothermal vents, however, cold seeps can occur at water depths of between a few metres and several kilometres, often along continental margins.
They are places where hydrocarbons – mostly methane but also ethane, propane, or even oil – seep from the sediment. Unlike at hydrothermal vents, the emanating fluids (gases and liquids) are no hotter than the surrounding seawater, and they are not necessarily under high pressure.
These hydrocarbons form up to several kilometres below the surface of the sediment when organic matter is degraded by either high temperatures or micro-organisms. When the hydrocarbons are produced in very large quantities, or where tectonic stress squeezes the sediments, the fluids rise to the sediment surface through cracks and fissures. The fluids may seep out at a continuous, low rate or the rate may vary.
Life at cold seeps: anybody home?
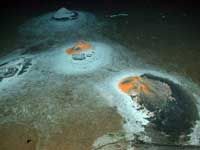
in an anoxic region, where
the oxygen level in the water
is too low to support
macrofauna
Image courtesy of MARUM,
Bremen University
Some fascinating creatures can be found at deep-water cold seeps, including giant tube worms, mussels, clams and crabs. How are these ecosystems supported?
Almost the entire seabed is home to micro-organisms. However, to support significant levels of microfauna in deep water, where the sunlight does not reach, requires oxygen-rich water and an alternative energy source – such as hydrocarbons.
One such energy source is methane, released at cold seeps. The anaerobic oxidation of methane (AOM) is a metabolic process with sulphate as the final electron acceptor, mediated by a symbiosis of methane-oxidising (methanotrophic) archaeans and sulphate-reducing bacteria.
CH4 + SO42- → HCO3- + HS– + H2O
AOM takes place in anoxic marine sediments, wherever methane from deep down and sulphate from the seawater meet. The end products of AOM, bicarbonate and sulphide ions, are released into the surrounding sediment and pore water (see glossary for all terms in bold).
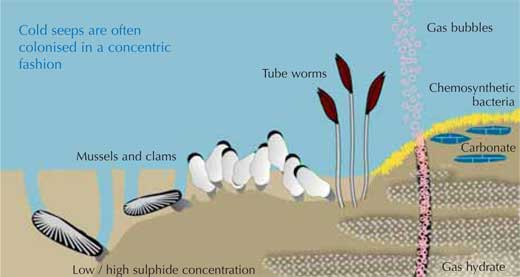
Image courtesy of BGR, after Sahling et al. (2002)
At locations where high methane concentrations are found close to the surface of the sediment (as in cold seeps), the sulphide produced by the micro-organisms can fuel an entire ecosystem. Microbial or faunal colonies at cold seeps can be between 100 cm2 and several hundred square metres in diameter. Where the sulphide-rich pore water escapes from the sea floor, the seep site will be colonised concentrically around these spots: closest to them will be those organisms which tolerate the highest concentrations of otherwise toxic sulphide (see image above).
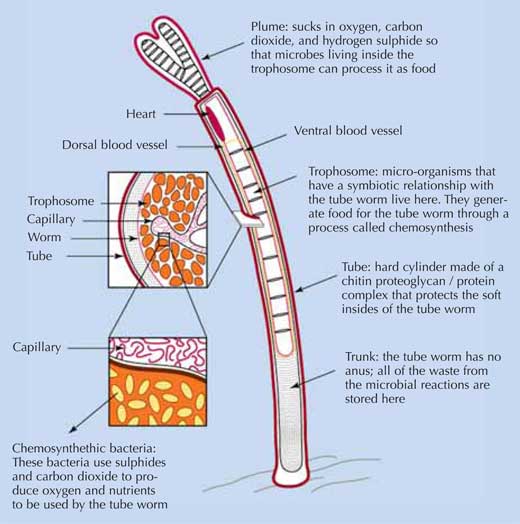
Image courtesy of Enduring Resources for Earth Science Education (ERESE)
At the basis of these ecosystems are methanotrophic and thiotrophic bacteria. Some of them live in chemosymbiosis with mussels (methanotropic bacteria), clams and tube worms (thiotrophic bacteria). The mussels and clams harbour the bacteria in their gills, whereas the tube worms shelter the bacteria in their trophosome; the bacteria, in return, supply their host with organic carbon (above).
The formation and fate of gas hydrates
Cold seeps are not only interesting because of the ecosystems they host: they could be important contributors to climate change and valuable new sources of hydrocarbons, to satisfy our increasing energy demands. Cold seeps generally indicate large amounts of hydrocarbons below the seabed, and they are comparably easy to identify because of their typical colonisation by specialised organisms.
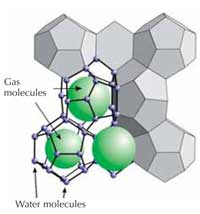
hydrates
Image courtesy of IfM-Geomar,
Kiel, Germany
Most interesting in this respect are gas hydrates. In these ice-like crystalline compounds, water molecules form a cage structure called a clathrate around individual gas molecules (see image on left) – in natural gas hydrates, this is mostly methane. Gas hydrates form where pore water is saturated with methane gas, within a narrow window of low-temperature and high-pressure conditions found only in deep permafrost soils – and in marine sediments at depths below about 400 m (see image below). At atmospheric pressure, gas hydrates are unstable and rapidly decompose into water and free gas.
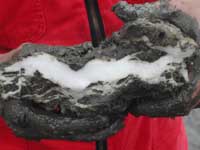
consolidate sea floor
sediments
Image courtesy of MARUM,
Bremen University
Gas hydrates store large amounts of chemically bound energy: because of the specific molecular structure, one litre of gas hydrate holds 0.8 l of water and 164 l of methane gas. The total energy resources in gas hydrates on Earth are estimated to be greater than those of all other known fossil fuels combined – ever.
Several countries, including the USA, Japan, South Korea, India and China, are exploring ways to harvest gas hydrates – safely, which is not a trivial matter. More importantly, however, it is essential to stop the gas hydrates from melting. Global warming is increasing the oceans’ temperatures, and this could cause a large-scale melting of gas hydrates in the sediment.
If they were to melt, the methane released into the atmosphere would react with atmospheric oxygen to form CO2, very efficiently enhancing the greenhouse effect. Left undamaged, gas hydrates act as stabilising agents for the continental slopes. If they were to melt, the slopes could destabilise, resulting in huge submarine landslides and tsunamis.
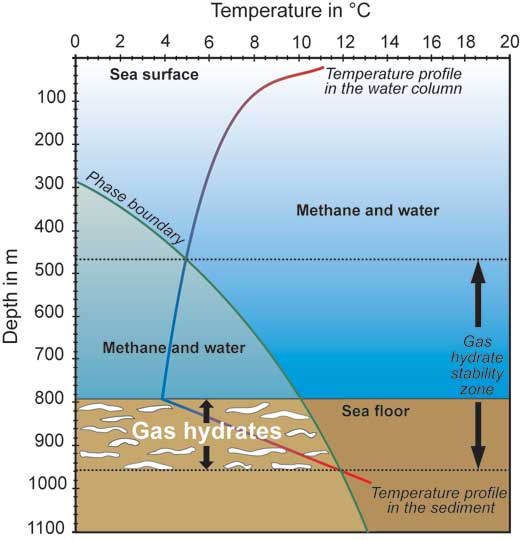
In the ocean, there are additional complications: in the water column, temperature decreases with increasing water depth, whereas in the sediment, temperature increases with increasing depth. The points at which these temperature profiles cross the theoretical phase boundary determine the depths at which gas hydrates may be found (the gas hydrate stability zone).
Furthermore, it is mostly in the sediment that methane concentrations are high enough to form gas hydrates (marked in white) – they have seldom been observed in the water column. Note that the scale in this diagram is an example and can vary depending on conditions
Image courtesy of David Fischer, MARUM
How do we study cold seeps?
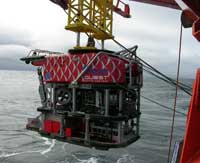
ROV suitable for depths of up
to 4000 m, equipped with a
large number of cameras,
spotlights, corers and other
tools to collect samples from
the sea floor as small as
single organisms only a few
millimetres long
Image courtesy of MARUM,
Bremen University
Studying cold seeps is obviously a big challenge for scientists: how can gases, water, sediment and organisms be sampled up to several kilometres below the sea surface? First, you have to reach your sampling location – this may take several days by ship, even for cold seeps on the continental shelf, and maintaining such a research vessel costs several tens of thousands of Euros a day. And when you get there, how do you reach the sea floor? Until the 1990s, the only way was to lower special tools on the end of a long steel cable, and recover them as soon as they had filled with sample material. This of course made it very difficult to have any visual control of where the tools hit the ground.
Matters have improved dramatically with the development of sophisticated underwater technology, such as remotely operated vehicles (ROVs) and autonomous underwater vehicles (AUVs), equipped with an array of cameras, lamps and sampling devices. MARUMw1 has its own workshop to develop such tools and robots for ocean research. Researchers use them to investigate cold seeps by either having them dive to individual spots to collect samples or taking photos of a larger area to provide an overall picture of a cold seep location.
There is still a lot of research to be done into the development and stability of cold seeps over time, and little is known about the organisms colonising them. Yet the most important question about cold seeps is how much methane is transferred into the ocean and subsequently into our atmosphere, where it will contribute to global warming. For me, it is very exciting to take part in this research.
Glossary
Chemosymbiosis: a symbiotic association between a multi-cellular organism (the host), which provides a protected environment, and a bacterium that oxidises specific chemicals to obtain energy and synthesise organic carbon that is required by the host
Methanotrophic: a methanotrophic organism metabolises methane as its only source of energy and carbon
Pore water: the water that fills the space between individual grains of sediment
Thiotrophic: a thiotrophic organism oxidises sulphur compounds
Trophosome: a specialised internal organ in tube worms, hosting symbiotic bacteria
Acknowledgements
The author would like to thank Dr Pape (MARUMw1) for valuable comments, particularly about gas hydrates. Moreover, Science in School and the author thank the publisher Inter-Research for permission to reuse the image from Sahling et al. (2002).
References
- Boetius A (2005) Microfauna-macrofauna interaction in the seafloor: lessons from the tubeworm. PLoS Biology 3(3): e102. doi: 10.1371/journal.pbio.0030102
- Little C (2010) Hot stuff in the deep sea. Science in School 16: 14-18. www.scienceinschool.org/2010/issue16/hotstuff
- Sahling H et al. (2002) Macrofaunal community structure and sulfide flux at gas hydrate deposits from the Cascadia convergent margin, NE Pacific. Marine Ecology Progress Series 231: 121-138. doi: 10.3354/meps231121
- The article can be downloaded free of charge from the Inter-Research website: www.int-res.com
Web References
- w1 – To learn more about MARUM – the Center for Marine Environmental Sciences, an independent DFG-funded research facility at the University of Bremen, Germany, see: www.marum.de
Resources
- MARUM offers a large selection of German-language resources and activities for teachers and school students, including videos and articles on research topics, a large selection of workshops for both primary- and secondary-school students in the MARUM teaching lab, workshops for primary-school teachers, experimental science theatre workshops for primary-school children, and much more. See: www.marum.de/en/entdecken.html
- MARUM has produced a wonderful (English-language) video on cold seeps and methane hydrates. See www.marum.de/marumTV.html
- In addition, MARUM has produced, in co-operation with the Deutsche Forschungsgemeinschaft, a 12-episode series of films about its research (DFG Science TV). Episode six (German only) tells the story of clams found at cold seeps. See http://www.marum.de/marumTV.html
- The US National Oceanic and Atmospheric Administration (NOAA) offers a number of lesson plans and downloadable teaching activities on cold seeps for all ages, plus background information. See the NOAA website (http://oceanexplorer.noaa.gov) or use the direct links:
- http://tinyurl.com/36zuhfb (‘Windows to the Deep’)
- http://tinyurl.com/32f6me6 (‘Expedition to the Deep Slope 2006’)
- http://tinyurl.com/35g3qrk (‘Expedition to the Deep Slope 2007’)
- For some general information about gas hydrates, see the MARUM website (www.marum.de) or use the direct link: http://tinyurl.com/3xzzpjj
- For more information on hydrocarbons, including gas hydrates, see: van Dijk M (2009) Hydrocarbons: a fossil but not (yet) extinct. Science in School 12: 62-69. www.scienceinschool.org/2009/issue12/energy
Review
If you are curious about the extremes of life on Earth, this is the article for you. David Fischer reports on exotic biological communities living at the bottom of the sea and on the exploration of these environments.
The language is easy enough to understand, with a glossary for technical terms. The article can be used in different subjects (biology, earth science, chemistry) to address a whole range of topics, such as: ecosystems, energy metabolism, food chains, natural resources, sedimentary rocks, non-renewable energy sources, marine environments and biodiversity, air pollution and the greenhouse effect, oceanographic research, hydrocarbons, clathrates, or redox reactions. Some of these topics (e.g. ecosystems, energy sources) are particularly suitable for an interdisciplinary approach.
The text also provides valuable background reading to introduce the origin of life at the bottom of the sea, for example with a comparison to hydrothermal vents.
In addition, the article provides references to content-rich websites (MARUM, NOAA) where the reader can find further information and resources (including teaching materials) on the topic. Therefore, I suggest completing the teaching unit with an activity from one of the cited web resources.
The article can also be used as a comprehension exercise. Possible questions include:
In AOM
- sulphate is oxidised to sulphide
- sulphide is reduced to sulphate
- methane is reduced to bicarbonate
- methane is oxidised to bicarbonate.
Gas hydrates
- consist of water molecules surrounded by gas molecules
- consist of gas molecules surrounded by water molecules
- are formed under high-temperature and low-pressure conditions
- are formed under high-temperature and high-pressure conditions.
Giulia Realdon, Italy