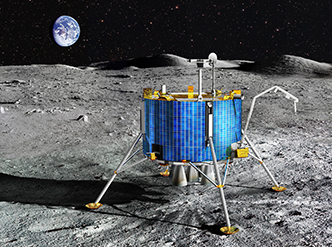
Landing on the Moon – planning and designing a lunar lander
Your mission: to land an intrepid egg-naut safely on the surface of the Moon and learn about classical mechanics along the…
A waste of space: years of human activity in space have left thousands of objects in orbit around the Earth. Learn more about the risks they pose and what we can do about it.
Our planet is surrounded by satellites, which we rely on every day for communication, navigation services, and Earth observation. For example, data from satellites can be used to support operations after disasters, monitor the status of large infrastructures, measure water and air pollution, and study climate change. While our societies are becoming more and more dependent on services provided from space, the satellites that we use for those services, and even the astronauts on board the space stations, are exposed to a growing threat from pieces of defunct satellites and rockets left in orbit. This is called space debris. We’ll explore what space debris is and where it comes from, the risk it poses to satellites in space and to future missions, and what can be done to mitigate this risk.
After over 60 years of space activity, more than 1000 objects larger than 1 m have been left in space. These are non-functioning satellites or parts of the rockets used to put them in orbit. Those objects tend to be concentrated in two specific regions of space: the so-called low-Earth orbit (LEO) and the Geostationary Orbit (GEO).
There are several different types of orbits, which have different characteristics and are useful for different purposes. Two very commonly used options are low Earth orbit (LEO) and geostationary Orbit (GEO).
LEO: these orbits are most commonly at altitudes of less than 2000 km and can even be as low as 160 km; most commercial aeroplanes do not fly any above around 14 km. Spacecraft in a LEO can circle the earth at any position, from around the equator to between the poles. They move at around 7.8 km per second and can circle the earth in around 90 mins.
GEO: this is a high-earth orbit with an altitude of 35 785 km. The speed of spacecraft at this altitude is just right to complete an orbit in exactly 24 hours, and the GEO also proceeds around the equator in the same direction as the spinning of the earth on its axis. This means that these spacecraft always remain above the same point on the Earth’s surface.
If we consider objects larger than 10 cm, the number of space debris objects is more than 30 000,[1] mostly consisting of fragments generated by almost 650 explosions and collisions occurring in space. Objects larger than 10 cm can usually be observed from the Earth and a catalogue of their position and velocity can be maintained, so that it is possible to predict their approximate future positions (blue in figure 1). This information is used by operating satellites to perform collision avoidance manoeuvres (CAMs) if one of these objects is along their path.
If we look at even smaller dimensions, it is estimated that there are around 1 million objects sized between 1 and 10 cm (red in figure 1). These are sometimes termed ‘lethal non-trackable’ objects as they are too small to be tracked from the ground but big enough to destroy a satellite in a collision. This is due to the fact that objects in orbit around the Earth have a very large velocity, in the order of 30 000 km/h in LEO. To understand how large this value is, we can imagine that if we were able to travel at that speed, we would go from Paris to New York in around 12 min. As the energy released in an impact is proportional to the square of the impact velocity, even small objects of 1 cm can still cause a significant amount of damage (figure 2). For comparison, the amount of energy released in the collision with such an object is comparable to that of a small car crashing into a wall at 40 km/h.
In the short term, space debris makes the operation of satellites more complex because of the need for monitoring debris and planning avoidance manoeuvres. While these manoeuvres are usually quite small, they still can cause an interruption of the service provided by the satellite, so this could be a problem if it started to affect a significant fraction of the operational time. In our current operations at ESA, we perform roughly one manoeuvre every three months for each satellite in the fleet, but what would happen if we would have to manoeuvre every month or every week?
In addition, not all the collisions can be avoided. Objects that are no longer functioning or don’t have a propulsion system cannot manoeuvre to reduce the risk. Furthermore, as mentioned above, most of the risk comes from objects that cannot be tracked and therefore avoided. For example, a solar panel on the Sentinel-1A satellite was impacted by an object just a few mm in size, creating a crater of 30 cm and generating new debris fragments (figure 3).
This is the main issue with space debris. Collisions can generate a large amount of new debris, which can trigger further collisions, and so on, with the potential for a domino effect called ‘Kessler’s syndrome’.[2] In this scenario, the number of space debris objects would grow so rapidly as to make some orbital regions practically unusable because of the high collision risk. Long-term simulations can be used to assess how far we are from such a situation. In these simulations, the evolution of the space debris population is forecast over 100 or 200 years, with assumptions on the number of launches in the future and the number of objects that are left inactive in orbit. The results indicate that continuation of our current behaviour in the future would lead to an unstable environment with collision rates increasing exponentially.[3]
Over 20 years ago, the technical community put forward a series of international guidelines covering different mitigation measures.[4] One of the key points is that spacecraft should leave their operational orbits once their mission is finished. For spacecrafts in LEO, the recommended action is to ensure that the satellite re-enters the Earth’s atmosphere within 25 years, whereas it takes far longer if the satellite is simply left in its operational orbit at altitudes above 600 km (figure 4). The idea is to exploit the residual atmosphere to have the spacecraft decay (i.e. reduce its altitude) and re-enter, burning up in the atmosphere. For GEO, where the effect of the atmosphere is negligible, the recommended action is to move the spacecraft into a so-called ‘graveyard’ orbit, which is high enough to ensure that they will not interfere with operational spacecraft for at least 100 years. However, although these guidelines are well known, the level of adoption is still insufficient[3] as they are usually adopted on a voluntary basis. Recently, new mechanisms to promote compliance with debris mitigation measures are emerging and, in October 2023, a first fine for a failed disposal from GEO has been issued in the US.
Another important mitigation measure is avoiding spacecraft explosions in space. This can happen when residual fuel is not disposed of. Of the nearly 650 debris-generating events known to date, those related to spacecraft propulsion systems have created the greatest amount of space debris (figure 5). For this reason, several technologies have been developed to empty fuel tanks at the end of a mission, for example, valves that can still work reliably after years in space. Similarly, methods are being developed to avoid battery explosions by effectively deactivating the power systems of a satellite.
Even if we stopped all future launches, long-term simulations of the debris environment[3] show that the population would still grow because of explosions and collisions of objects already in orbit. For this reason, active debris removal (ADR) missions are under development worldwide. The purpose is to launch a satellite equipped with specific capturing systems (e.g., robotic arms, harpoons, or nets[5]) to remove non-functioning spacecraft from orbit and promote re-entry. While some demo missions have already been carried out,[6] the first mission to remove an item of debris from orbit is currently planned for 2025.[7] An artistic representation of the mission is shown in figure 6.
In conclusion, as on Earth we are becoming more and more aware of the issue of sustainability of our activities, the same applies to space. Limiting the production of space debris and making sure that space missions follow guidelines for debris mitigation is a necessary step to enable future generations to continue to have access to space and collect the important benefit of earth-orbiting satellites.
[1] ESA, Space debris by the numbers: https://sdup.esoc.esa.int/discosweb/statistics/
[2] Kessler DJ, Cour-Palais BG (1978) Collision Frequency of Artificial Satellites: The Creation of a Debris Belt. Journal of Geophysical Research 83: 2637–2646. doi: 10.1029/JA083iA06p02637
[3] The ESA’s Annual Space Environment Report (2023): https://www.sdo.esoc.esa.int/environment_report/Space_Environment_Report_latest.pdf
[4] IADC Space Debris Mitigation Guidelines Rev. 3 (2021): https://www.iadc-home.org/documents_public/file_down/id/5251
[5] Biesbroek R et al. (2013). The e.Deorbit CDF Study: A Design Study For The Safe Removal Of A Large Space Debris. Proceedings of the 6th European Conference on Space Debris: https://conference.sdo.esoc.esa.int/proceedings/sdc6/paper/122/SDC6-paper122.pdf
[6] Aglietti GS et al. (2020). The active space debris removal mission RemoveDebris. Part 2: In orbit operations. Acta Astronautica 168 310–322. doi: 10.1016/j.actaastro.2019.09.001
[7] Biesbroek R et al. (2021) The ClearSpace-1 mission: ESA and ClearSpace team up to remove debris. Proceedings of the 8th European Conference on Space Debris: https://conference.sdo.esoc.esa.int/proceedings/sdc8/paper/320/SDC8-paper320.pdf
The development of science has also meant the conquest of space. Man sends satellites into space to investigate it, but also for the many applications of these satellites in everyday life: global communications, weather forecasting, tracking fires, floods etc. The appearance of space debris creates problems for the future. That’s why it’s so important to know how to minimise their alarming growth by reading this article.
Corina Toma, Physics teacher, Romania
Your mission: to land an intrepid egg-naut safely on the surface of the Moon and learn about classical mechanics along the…
What would the world look like if we could see infrared light? With some simple modifications, you can turn a cheap webcam into an infrared camera…
We can’t image our home galaxy from the outside, so how do we study it? Learn how astronomers unveil the dramatic past of the Milky Way and peer into its future.