When things don’t fall: the counter-intuitive physics of balanced forces Teach article
Intrigue your students with some surprising experiments – it’s a great way to challenge their intuitions and explore the laws of mechanics.
Why do we teach science? Is it to give students new ideas – or to remove ideas they already hold? In some areas, such as the physics of free fall, it seems the latter is the real task: while the ideas described by Newtonian mechanics are simple enough, our intuitions can make them difficult to accept.
When students arrive at physics classes, they already possess firm ideas about many physical situations. Research in science education has shown that students often hold a set of intuitive rules about the mechanisms underlying physical changes (Viennot, 2014). Some of the most widely held of these rules include:
- The greater the cause, the bigger the effect.
- Several agents have a greater effect than just one.
- If there is a cause, an effect must follow.
In addition, according to our findings (Tsakmaki & Koumaras, 2014; Tsakmaki, 2016), students seem to follow what is known as ‘the rule of type’: the cause and the effect are both of the same type.
We use the term ‘type’ here to mean any of the following:
- The spatial orientation of cause and effect
- The kind of change (such as an increase or decrease)
- The intensity of the cause and the effect.
In this article, we analyse three simple experiments that involve the rule of type. They can be used to reveal how students reason and to enable teachers to present physics concepts in a more accessible way.
The first experiment is an extension of the popular textbook problem that asks students to draw the forces acting on a coin tossed straight up into the air. Students have difficulty realising that (if we ignore air resistance) once the coin has left the hand, the only force acting on it is its weight. In fact, the coin is in free fall while going up! Replacing the coin with water demonstrates this fact beyond doubt.
The other two experiments explore ideas of balance: the second using a pulley, and the third using buoyancy. All the experiments are suitable for science students aged 14–16.
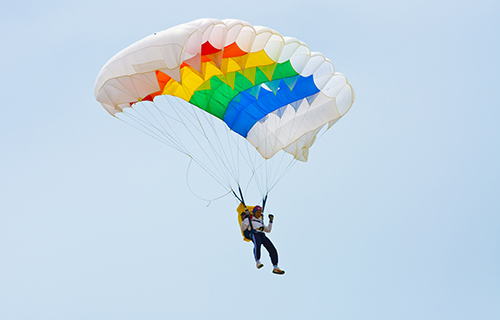
Image courtesy of hinnamsaisuy / shutterstock
Experiment 1: weightless water
This is a brilliant experiment to demonstrate weightlessness. We suggest doing this as a teacher demonstration (rather than student experiment). Allow 30 minutes for the experiment and discussion, plus 10 minutes for preparation.
Materials
- One small plastic bottle (e.g. 500 ml or 750 ml soft-drink bottle)
- Tap water
- Location where water spillage is not a problem (e.g. outdoors)
- Smartphone with slow-motion filming capability (optional)
Procedure
- Pierce both the base and the cap of the plastic bottle.
- Fill the bottle with water. Place a finger on the hole in the cap to show that the water stops escaping from the base.
- Now remove your finger from the cap to show that the water flows out of the hole in the base (figure 1).
- Ask students to predict how water flow will be affected if we let the bottle fall freely. (Students usually predict correctly that the water will stop flowing out while falling.)
- Refill the bottle. Test the prediction by letting the bottle fall from shoulder height (figure 2). (If possible, film this using the slow-motion option on a smartphone, so that students can review exactly what happens.)
- Ask students to predict what will happen if we throw the bottle upwards. Will water flow out or not?
- Refill the bottle and throw it upwards (figure 3), while the students watch (or film it as before) – and then discuss what happened.
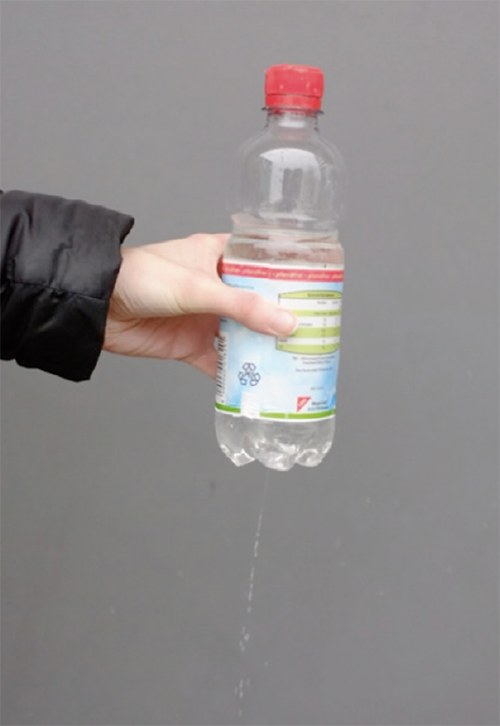
Image courtesy of Spilios Vasilopoulos
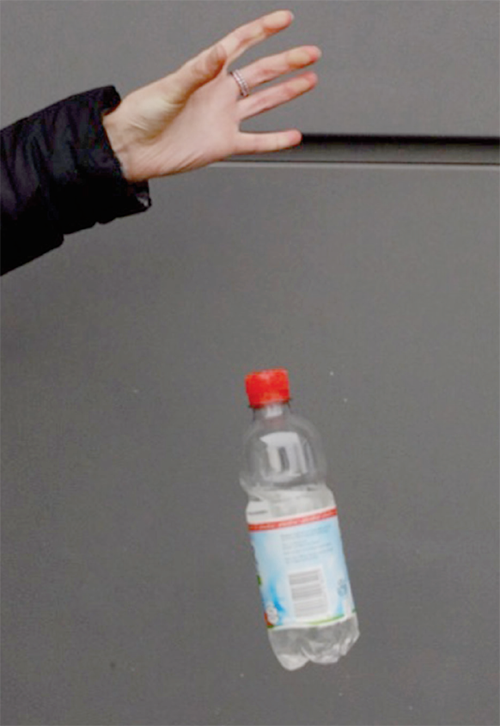
Image courtesy of Spilios Vasilopoulos
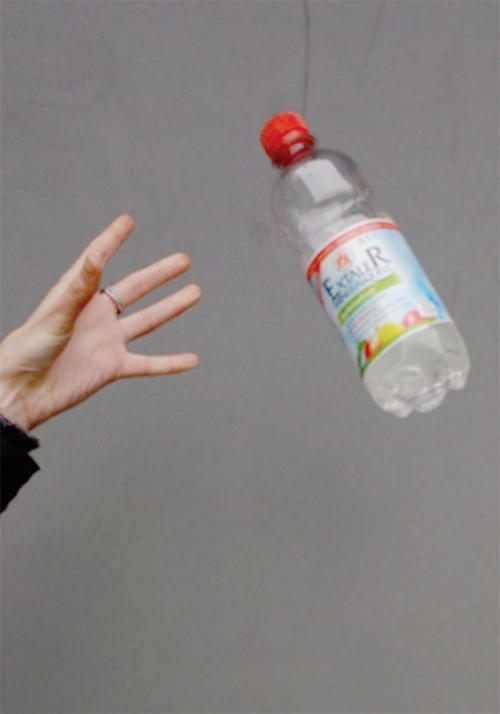
Image courtesy of Spilios Vasilopoulos
Discussion
When the bottle is at rest, water flows out through the hole in the base. This is consistent with the rule of type: the cause (the water’s weight) is directed downwards, and the effect (the downward flow of water out of the bottle) is similar. Most students correctly predict that when the bottle is falling, the outflow of water will be ‘cancelled’ during the fall because both the bottle and the water are moving downwards in the same way.
But what happens when the bottle is thrown upwards: will water still flow out? Most students (more than 80% of ours) think it will. Their reasoning is often based on what we experience when standing in a lift (Corona et al., 2006). As it moves upwards, we feel heavier, so the water should ‘feel heavier’ and thus flow out faster.
This prediction is wrong. As the demonstration shows, no water flows out during the upwards or downwards motion of the bottle. After the bottle leaves the hand, the only force acting on it is its own weight, so it is in free fall – even when the bottle is moving upwards (because it is decelerating). Like orbiting astronauts, the water in the bottle ‘feels’ weightless – and no weight means no water flowing out.
The students’ erroneous reasoning is in line with the rule of type: here, the imagined cause is the supposed increase in the water’s weight due to being launched upwards, so the effect should be an increase in water flowing out. The experiment shows that what happens is the opposite of what’s expected, challenging the students’ intuitive reasoning.
Experiment 2: asymmetrical balance
In this experiment, two weights are balanced – but, unlike with a set of balance scales, they are not on the same horizontal level. Allow 20 minutes for the experiment and discussion, plus 10 minutes for preparation.
Materials
- Block of wood, about 10 cm x 10 cm x 10 cm (or similar volume)
- Small bucket with handle
- Dry sand (amount similar in mass to the wood block)
- Pulley (single wheel and cord)
- Balance or scales to weigh the wood block, bucket and sand
Procedure
Steps 1–4 are preparation; the demonstration is steps 5–6.
- Weigh the wood block.
- Add dry sand to the bucket until the bucket plus the sand weigh the same as the wood block.
- Set up the pulley so that the cords are approximately the same length on each side of the wheel. Attach the wood block to one side and the bucket of sand to the other.
- Ensure the wood block and bucket of sand are exactly balanced so that neither falls down. Adjust the amount of sand if needed.
- Starting with the block and bucket in level positions, show the set-up to the students (figure 4). Then, move the wood block upwards so that the bucket is now lower than the block. While holding the wood block, ask the students to predict what will happen if you let go of it.
- Let go of the wood block. What happens? Nothing! Ask the students to explain this.
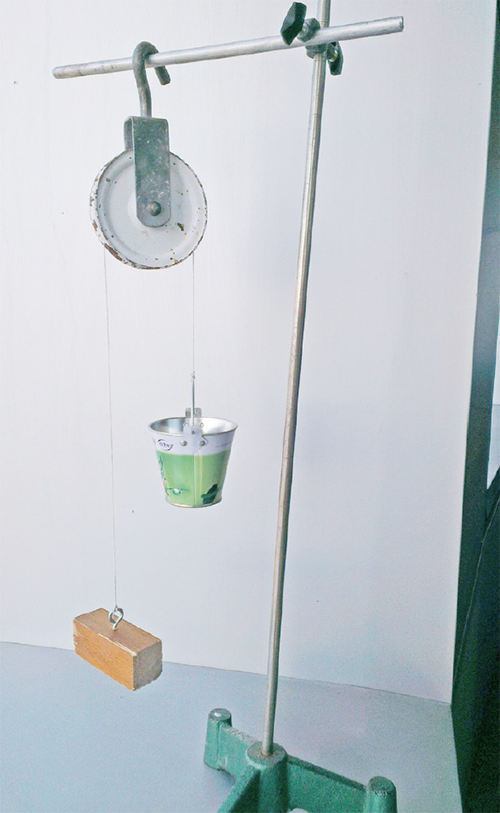
Image courtesy of P Koumaras
Discussion
When we ran this experiment, almost 50% of our secondary school students predicted that the raised block would return to its initial position – despite the fact that if the two objects are in static equilibrium, the net force acting on each object is zero and the block will not move.
When students see the block and bucket at different horizontal levels, they seem not to notice that the two objects are balanced. Instead, they focus on the most striking observable feature of the situation: the different heights of the two objects. This appears to represent an effect – so, in line with the rule of type, there must be a cause of the same type: unequal forces. As a result, many of the students conclude that one object (the one nearer the ground) must be heavier than the other one.
Experiment 3: floating under water
Materials
- Plastic bottle (e.g. 500 ml soft-drink bottle) with cap
- Tank (ideally a tall column shape) filled with water
- Sand
- Force meter
- Some cord
Procedure
Steps 1 and 2 are preparation; the demonstration is steps 3–6.
- Fill the bottle with water.
- Add a small amount of sand to the bottle and replace the cap. Adjust the amount of sand until the bottle does not move up or down when placed at any level in the tank (figure 5).
- Initially, place the bottle close to the bottom of the tank.
- Then, move the bottle close to the water’s surface. Ask the students to predict in which of the two places the buoyancy acting on the bottle is greater.
- Using the cord, connect a force meter to the bottle. This measures the net force on the bottle: weight minus buoyancy. (When moving the submerged bottle to the higher level, move the force meter gently upwards with it so that it does not pull on the bottle.)
- Read the meter with the bottle in both places: it will show a force of zero.
- Ask the students to explain this.
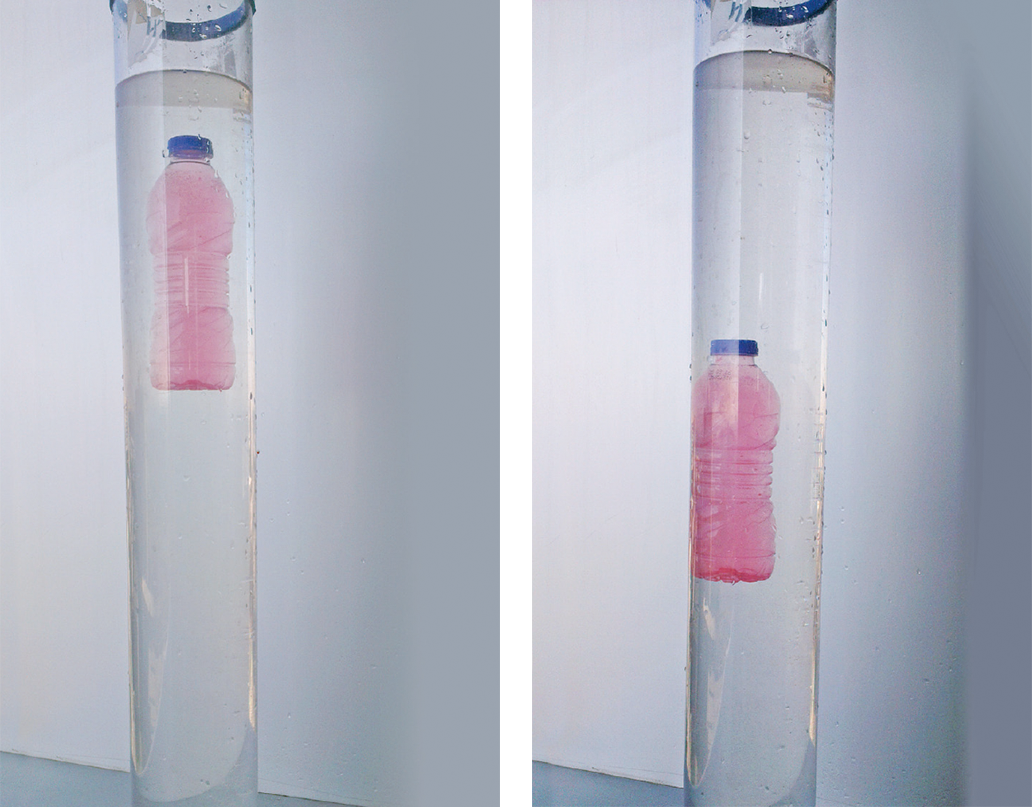
Images courtesy of P Koumaras
Discussion
When we ran this experiment, more than 40% of our students predicted that the higher the bottle is in the tank, the greater the buoyancy acting on it. In fact, the bottle is in static equilibrium in both positions, so the net force acting on it is zero.
When students see the bottle floating at different levels, some of them seem to ignore the fact that the forces on it are balanced. As in the previous experiment, they focus instead on what they see – unequal levels – and infer that there must be a cause of the same type: unequal forces. The weight of the bottle is the same throughout, so they conclude that when it is higher up, it must have greater buoyancy.
End note
We believe that these counter-intuitive demonstrations are more than just curiosities: we can use these mismatches between intuition and experiment in the classroom to help understand students’ misconceptions. And by integrating these experiments into teaching in a structured way, we can provide some real benefits for learning.
References
- Corona A, Slisko J, Planinsic G (2006) Freely rising bottle of water also demonstrates weightlessness. Physics Education 41(3): 208-209
- Tsakmaki P (2016) Looking for causal ‘laws’ on students’ prediction and interpretation of physical phenomena and didactical utilization of them in physics’ instruction. Unpublished PhD thesis, School of Education, Aristotle University of Thessaloniki, Greece (under consideration)
- Tsakmaki P, Koumaras P (2014) Causal rules: what might be hinting in misconceptions of our students? 8th Hellenic Conference on History, Philosophy and Didactics of Science, Patra 14–16 October 2014 [in Greek]
- Viennot L (2014) Thinking in Physics: The Pleasure of Reasoning and Understanding. Dordrecht, the Netherlands: Springer. ISBN: 9789401786652. doi: 10.1007/978-94-017-8666-9
Resources
- These short videos demonstrate the three experiments in the article:
- For another article about counter-intuitive effects in mechanics, see:
- Norrby M, Peltoniemi R (2016) Can something accelerate upwards while falling down? Science in School 37: 25-28.
Review
This article introduces the not-so-simple topic of so-called ‘counter-intuitive experiments’ in physics and describes how students use the ‘rule of type’ to explain the results of the experiments.
Getting feedback from students on the way they think and how they rely on preconceived ideas is a useful outcome for teachers.
The teaching activity seems to be useful mainly for teaching physics to students aged 14-16.
The article encourages teachers to ask questions such as:
- What happens to the water if the bottle is moved up or downwards – and why?
- Do the effects depend on the speed of the movement?
- How would you describe the concepts of gravity, mass and weight?
Ged Vogt, Higher Secondary School for Environment and Economics, Yspertal, Austria