Greening chemistry Understand article
Chemistry is not always completely environmentally friendly; green chemistry is working to change that.
Chemistry has a mixed reputation. We all benefit from the consumer goods and medicines that the chemical industry produces, but there is also a dark side – such as the industry’s huge power needs and its creation of toxic solvents, reactants and waste products. For more than 20 years, chemists have been trying to clean up chemistry through the growing field of green chemistry.
Also known as sustainable chemistry or environmentally benign chemistry, green chemistry is a concept that is slowly being introduced at school level. Two of its earliest champions, Paul Anastas and John Warner, define green chemistry as ‘the design of chemical products and processes that reduce or eliminate the use and generation of hazardous substances’.
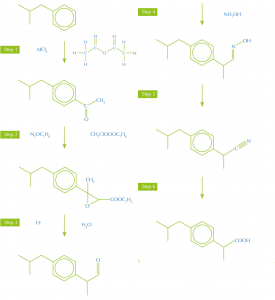
The removal of, or reduction in, chemical waste; the lowering of total energy requirements for chemical processes; and an increase in safety awareness are in the interests of humanity and the environment. Industry too is interested; a report in 2011 suggested that green chemistry could save the industry US$65.5 billion by 2020.
The main ideas underlying green chemistry can be summed up briefly in a few simple points that could form interesting topics for general class discussion or an in-depth consideration of the chemical processes traditionally taught in schools. Here we will look at this from a climate chemistry perspective. A full list of the 12 principles of green chemistry can be found onlinew1, but we will limit ourselves to those aspects that are more applicable to school-level chemistry: atom economy, the source of the reactants, using environmentally benign substances and reducing energy use.
Reducing waste
One theme of green chemistry is how much of the reagents and solvents end up in the desired products, rather than as waste or by-products. Reducing the waste reduces the associated environmental problems. The idea of ‘atom economy’ and the simple exercises that students can perform (see box) will underpin such ideas. The sources of starting materials should also be considered. Reactants should be renewable wherever appropriate or obtained from sources that are readily and easily available.
Pharmaceuticals, in particular, are often produced in a multi-step synthesis rather than simply by reacting reagent A in a test tube with reagent B to create the drug. In a multi-step synthesis, the product made in one step is used in the next but the yield of each step is much less than 100% so materials, solvents and energy are lost along the way.
Synthetic routes with fewer and more productive steps, bearing in mind the other green principles, make less waste. For example, the painkiller ibuprofen was originally made in a six-step synthesis from the starting material isobutylbenzene, but a new, more efficient, synthesis only uses three steps (figure 1).
Reducing toxicity
Green chemists also try to use only substances that do not harm the environment. In one of the world’s worst industrial disasters, methyl isocyanate gas (CH3NCO) used in pesticide production leaked from the Union Carbide India Limited plant in Bhopal, India, in 1984. Thousands of people were exposed. The immediate death toll was reported as several thousand, and around half a million people were injured, suffering temporary or permanent disability.
As well as choosing less toxic reagents, green chemists also try to switch to non-organic solvents like water and super-critical carbon dioxide. The previously used hydrocarbon solvents are toxic and emit vapours that are greenhouse gases, while halogenated solvents are often carcinogens as well as greenhouse gases and sources of free radicals –which destroy ozone.
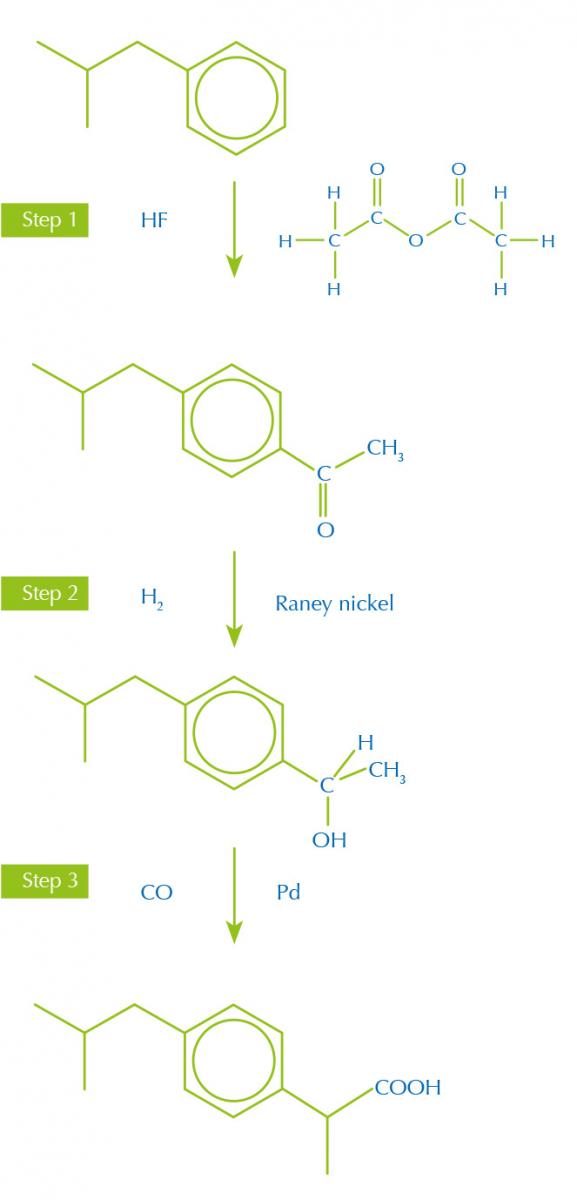
synthesis of ibruprofen
Image courtesy of Nicola Graf
Reducing emissions
The chemical industry needs a huge amount of electricity; reducing that requirement is important both economically and environmentally. Researchers use energy to heat and increase the pressure of reactions as well as to transport materials. Scientists are looking for alternative reaction pathways that work at lower temperatures and pressures, reducing the amount of carbon dioxide produced by the burning of fossil fuels.
Finding more efficient catalysts for existing reactions will also cut down on reactant and energy waste. Ideally, catalysts would use readily available metals such as iron rather than more exotic ones such as platinum, which are scarce and require considerable energy to obtain. Some catalysts also become ‘poisoned’ during the reaction, meaning that chemicals bond to the active sites of the catalyst and form products on the surfaces that may be toxic and difficult to dispose of safely.
Applying green chemistry to green fuels
When energy is needed, biofuels are desirable substitutes for fossil fuels. Ethanol is a well-known biofuel produced by many fermentation processes. Ethanol can be produced from vegetable matter including some grasses and corn husks. Adapted vehicles can use 100% ethanol (E100) or ethanol–petrol mixtures, known as gasohol, ranging from E5 to E25. However, ethanol is not a particularly good fuel as it:
- does not vaporise as easily as gasoline
- has only around 70% the energy density of gasoline
- has a tendency to attract water
- is acidic at the temperatures within the engine and can cause corrosion
- requires engines to be adapted to run with larger proportions of it.
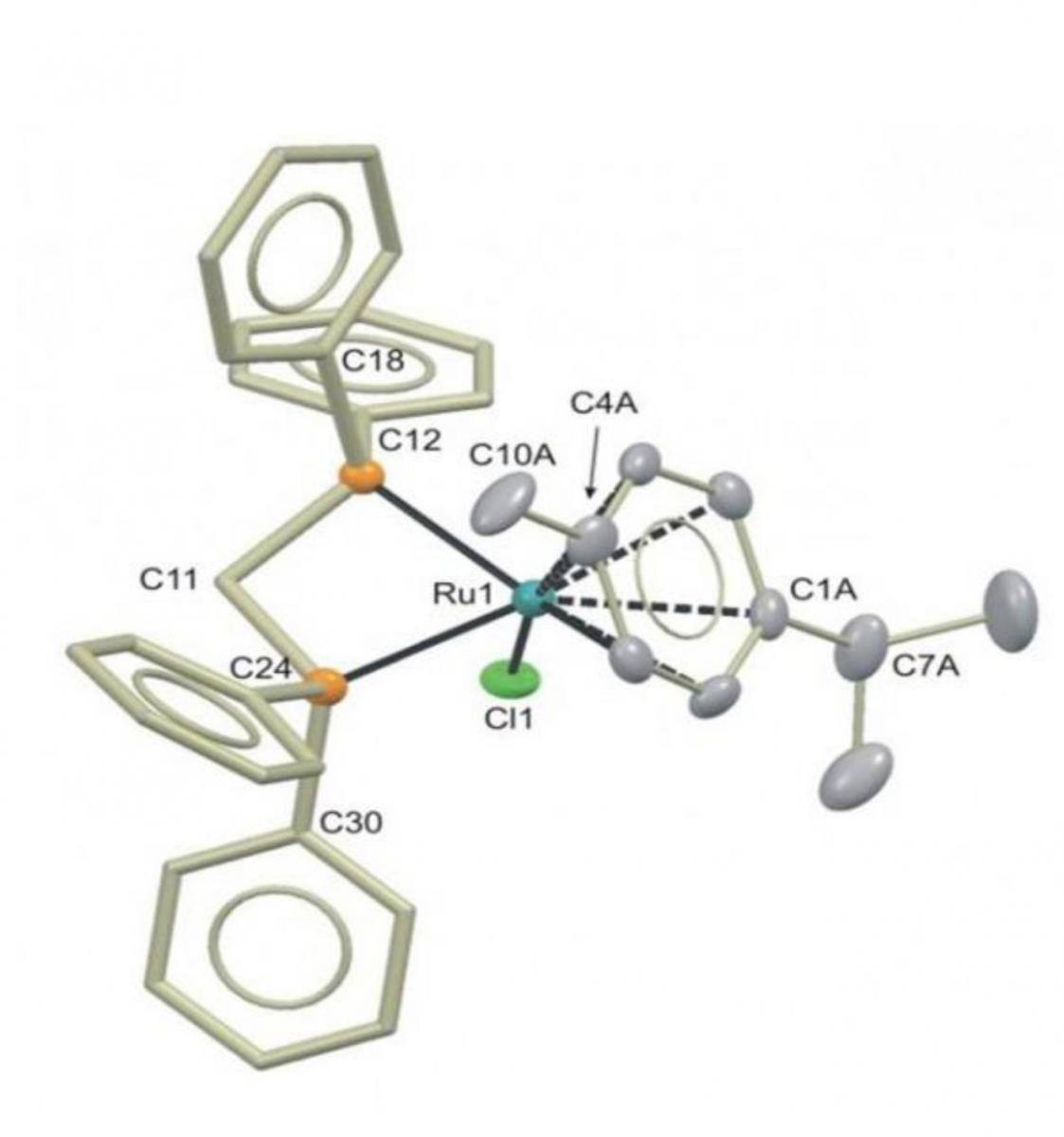
catalyst used to make
butan-1-ol from ethanol
Image courtesy of Duncan
Wass University of Bristol
Butan-1-ol (which has an energy density of 29.2 MJ/l) would be a much better fuel additive than ethanol (19.6 MJ/l) as it has properties more similar to petrol (32.0 MJ/l). Butan-1-ol can be produced from biomass (biobutanol) and from fossil fuels (petrobutanol). However, these processes either need large amounts of energy or rely on biological catalysts (enzymes) and produce low yields. The separation of butan-1-ol from a mixture of products is also energy demanding.
It has long been the target of the chemical industry to make butan-1-ol by condensing two ethanol molecules together:
CH3CH2OH + HOCH2CH3 → CH3CH2CH2CH2OH + H2O
In 2013, however, a breakthrough enabled butan-1-ol to be produced from ethanol in yields of more than 95% using a ruthenium catalyst. The challenge now is scaling the process up for industry.
Environmental problems are not going away, so green chemistry will become even more important. Bright young people will be needed to apply green chemical principles throughout chemical sciences and engineering.
Atom Economy
Traditionally, chemists have calculated the efficiency of a chemical reaction through a percentage yield calculation.
Percentage yield = (moles of product achieved / moles of product expected) x 100
Barry Trost of Stanford University, USA, introduced the concept of atom economy as an alternative way to view the efficiency of a reaction. Atom economy relates the total mass of starting materials to the mass of desired product. Therefore, the calculation highlights the wasted resources: if little of the starting materials are in the product, they must be in the waste.
Percentage atom economy = (mass of desired product / total mass of all reagents) x 100
It is easy to demonstrate by calculation that a chemist using atom economy would use simple starting materials to build up a product rather than break a large molecule down, thereby producing a lot of waste products.
Consider the reactions in which magnesium oxide is the desired product:
2Mg + O2 → 2MgO
2 x 24 = 48 g 32 g 2 x 40 = 80 g
Atom economy = (80 / (48 + 32)) x 100 = 100%
MgCO3 → MgO + CO2
84 g 40 g 44 g
Atom economy = (40 / 84) x 100 = 48%
More advanced students may like to look at how changes to the production of ibuprofen would improve atom efficiencyw2.
References
- Anastas PT, Warner C (1998) Green Chemistry Theory and Practice. New York, NY, USA: Oxford University Press. ISBN: 0198502346
- Trost BM (1991) The atom economy – a search for synthetic efficiency, Science 254: 1471–1477
Web References
- w1 – The 12 principles of green chemistry can be found on the website of the American Chemistry Society.
The ACS website also offers a range of educational resources on green chemistry, including books, online resources and experimental protocols for all age groups (from secondary school to undergraduate). - w2 – The Royal Society of Chemistry’s Learn Chemistry website has a lesson on the synthesis of ibruprofen, which also considers the drug’s structure and bonding.
Resources
- For practical activities associated with climate change, please see:
- Shallcross D, Harrison T (2008) Practical demonstrations to augment climate change lessons. Science in School 10: 46–50.
- Shallcross D, Harrison T, Henshaw S, Sellou L (2009) Fuelling interest: climate change experiments. Science in School 11: 38–43.
- Shallcross D, Harrison T, Henshaw S, Sellou L (2009) Looking to the heavens: climate change experiments. Science in School 12: 34–39.
Review
As the authors point out, chemistry is surrounded by a negative halo among students and general public, but the chemistry behind objects and materials is indispensable in our life. This is the reason why I recommend this article for use before addressing a chemistry course in secondary school.
The ideas behind green chemistry are clearly outlined in a simple style with examples taken from everyday life, from drug synthesis to biofuels. The topic, of course, would be worth further deepening and, if possible, direct experience through practical activities.
Both these objectives are at hand by means of the quoted references (ACS website and Royal Society of Chemistry educational pages).
Moreover, the history of chemical accidents, such as the Bhopal disaster, can provide teachers with elements for discussing chemical safety, environmental sustainability and the role of green chemistry.
Gulia Realdon, Italy