Peering into the darkness: modelling black holes in primary school Teach article
Having difficulties explaining black holes to your students? Why not try these simple activities in the classroom?
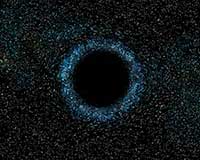
impression of a black hole.
The black hole is only a point
in the very centre, but its
gravity is so strong that the
light from stars around
it is unable to escape.
Image courtesy of the
European Space Agency,
NASA and Felix Mirabel (the
French Atomic Energy
Commission & the Institute for
Astronomy and Space Physics /
Conicet of Argentina)
Many young people have heard of black holes and understand that if something falls into one, it cannot get out again – even light cannot escape. That is how a black hole gets its name: it is a point in space that does not emit any light (figure 1). This is not an easy concept to explain. In this article, therefore, I briefly introduce black holes and then describe two simple activities to help school students to visualise what is happening. Each activity should take about an hour; both are suitable for pupils aged 10-14 (although note that the reviewer suggests using the activities with students aged 10-19).
Black holes
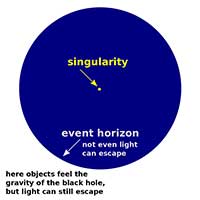
collapsed star or singularity;
the event horizon, a region
around the singularity where
not even light can escape;
and the region outside the
event horizon, where objects
can feel the gravity of the
black hole without becoming
trapped. Click on image to
enlarge.
Image courtesy of Monica
Turner
Black holes form during the death of very massive stars (at least several times the mass of our Sun).
A star consists of a hot core surrounded by many layers of gasw1. In the core of the star, lighter elements such as hydrogen and helium are joined together by thermonuclear fusion to form heavier elements such as metals. The heat created in this process exerts an outward pressure, which counteracts the force of gravity pulling the gas towards the centre of the star and gives the star its large size. When the star runs out of fuel in its core, however, it is unable to support these heavy outer layers of gas. If the dying star is very massive, gravity will pull on the gas and cause the star to become smaller and smaller until its density reaches infinity at a single point, which is called a singularity (figure 2).
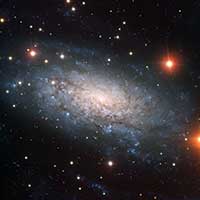
3621, taken using the Very
Large Telescope at the
European Southern
Observatory (ESO). This
galaxy is believed to have an
active supermassive black
hole at its centre that is
engulfing matter and
producing radiation.
Image courtesy of ESO
Close to the singularity, gravity is so strong that nothing can escape. The escape velocity would need to be higher than the speed of light – so not even light can escape, which is why the black hole is black. (It is not actually a hole, though: there is a lot in there, although we cannot see it.)
At a certain distance from the singularity, gravity is weak enough to allow light to escape, thus objects beyond this distance are visible. This boundary is called the event horizon. Objects outside the event horizon still feel the black hole’s gravity, and will be attracted towards it, but they can be seen and can potentially escape falling in. Once objects are sucked inside the event horizon, however, there is no return.
After the black hole forms, it can grow by absorbing mass from its surroundings, such as other stars and other black holesw2. If a black hole absorbs enough material, it can become a supermassive black hole, which means it has a mass of more than one million solar masses. It is believed that supermassive black holes exist in the centres of many galaxies, including the Milky Way.
Usually, astronomers observe objects in space by looking at the light; this, for instance, is how they study stars (for example, see Mignone & Barnes, 2011). However, since black holes do not emit any light, they cannot be observed in the usual way. Instead, astronomers have to observe the interaction of the black hole with other objects. One way to do this is to look at the motions of stars around the black hole, since their orbits will be altered by its presencew3.
Activity 1: Modelling the formation of a black hole
This activity will demonstrate to students how a black hole is formed through the collapse of a massive star, once the core of the star is unable to support the weight of the outer layers of gas surrounding it. The time needed should be about one hour.
Materials
Each working group will need:
- A balloon
- A few sheets of aluminium foil, each approximately 30 cm square
- A pin for popping the balloon.
Method
- Have the students inflate the balloon and tie it closed. They should then wrap the balloon in several layers of aluminium foil to create the model star.
- Explain that the layers of foil represent the different gas layers of the star, and the balloon that gives them their shape is analogous to the hot burning core of the star. Inside the core, the heat created by thermonuclear fusion exerts a pressure on the gas layers of the star, which keeps them from collapsing.
- Have the students simulate the effect of gravity by trying to lightly compress the balloon. The pressure of the core is such that the star cannot collapse from gravity.
- When a star reaches the end of its life, it runs out of fuel in the core and is no longer able to hold up the gas layers. Have the students pop the balloon with the pin, which simulates this process.
- Again, they should try to compress the balloon with their hands to mimic the effect of gravity. This time, they will be able to compress the foil into a small ball, which simulates the formation of a black hole. Note that the mass of the small ball is the same as that of the model star, but their sizes are quite different.
Discussion
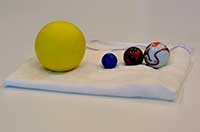
required for the activity 2
Image courtesy of Charlotte
Provost and Monica Turner
- If a real star were the size of the balloon, then how big would the black hole really be? Is the crumpled ball too large or too small to represent a real black hole? Answer: The crumpled ball is much too large to represent a black hole. Even a real black hole, formed from a massive star, is smaller than the tip of a pencil.
- What would happen if you used more pieces of aluminium foil to make the gas layers in the star? Would the star be more massive? What about the black hole?
Building the star with more layers of gas (represented by the foil) would make the star more massive. It would also result in the formation of a more massive black hole, since there would be more material with which to form the black hole.
- The concept of density (mass per unit volume) could be introduced here. Which has a higher density, the star or the black hole? Although they have a different size, the star and black hole have the same mass, since they are made from the exact same amount of material. However, since the black hole is smaller, it has more material contained in less volume, and therefore has a higher density.
Activity 2: Modelling the action of a black hole
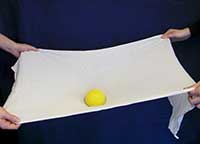
in the centre causes the
space-time fabric to curve.
Image courtesy of Charlotte
Provost and Monica Turner
In this activity, students will build a model of a black hole to help them visualise how a black hole can ‘bend’ space–time and affect nearby objects. The activity should take about one hour.
Materials
Each working group will need (figure 3):
- A light elastic bandage used for muscular injuries (e.g. Tubifix, sold in chemists’ shops), the largest ones available (used for the thorax)
- A small marble
- A very heavy ball (such as those used in games of boules, bocce or pétanque)
- A pair of sharp scissors.
Method
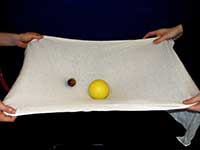
along the fabric, and observe
how it trajectory is altered.
Image courtesy of Charlotte
Provost and Monica Turner
- Cut a piece of elastic bandage about 40 cm long. If it is tubular, you will need to cut it open on one side.
- Ask several students to stretch the bandage horizontally until it becomes taut, to represent two-dimensional space.
- Place the marble on the bandage, and make it roll across the surface of the bandage. Its path should be a straight line, similar to that of a light ray travelling through space.
- Place the heavy ball on the bandage, and you will see how it deforms the fabric of space. Space becomes curved around the heavy mass.
- Make the marble roll close to the mass; its trajectory should be altered by the deformation of the bandage. This is similar to what happens to light passing close to a massive object that deforms the space surrounding it. Try varying the speed of the marble to see how its path changes.
- The more concentrated the central mass (that is, the heavier the large ball), the more curved the bandage will be. This increases the depth of the ‘gravitational well’, from which a marble would not be able to escape.
- As the marble passes close to the large ball, it starts to revolve around the ‘black hole’ and eventually falls in. Once it is there, you can see how things may easily fall into a black hole but have difficulty getting out. This is what happens with black holes: their gravity deforms space in such a way that light or other objects fall in and cannot escape.
Discussion
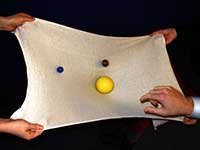
different weights.
Image courtesy of Charlotte
Provost and Monica Turner
- What happens when you decrease the speed of the marble? Why? When the speed of the marble is high enough, the marble has enough energy to escape the gravity of the black hole. However, if the speed of the marble is too low, the force of gravity from the black hole is too strong and the marble will not be able to escape.
- What happens when you use a heavier large ball? What about a heaver marble (figure 6)? Because more massive objects create a stronger gravitational force, in both cases you will need to throw the marble harder for it to escape the gravity of the black hole.
- How would you be able to tell if there is a black hole somewhere by observing the motions of the stars? If a black hole becomes massive enough, stars that pass nearby will become trapped in its gravitational field and begin to orbit the black hole, much as the planets in our Solar System orbit the Sun. By observing the motions of many stars, astronomers can look for stars that have orbits around the same central point. If they cannot see an object at this central point, this is evidence that a black hole could be present there.
Acknowledgements
Activity 1 was adapted from the ‘Journey to a Black Hole’ demonstration manual on the Inside Einstein’s Universe websitew4. That activity was in turn adapted from the ‘Aluminum Foil, Balloons, and Black Holes’ activity on NASA’s Imagine the Universe websitew1.
Activity 2 is adapted from a resource in the UNAWE database by Ricardo Moreno from Exploring the Universe, UNAWEw5 Espagna.
References
- Mignone C, Barnes R (2011) More than meets the eye: the electromagnetic spectrum. Science in School 20: 51-59.
Web References
- w1 – NASA’s Imagine the Universe website provides information for both teachers and students about the life cycles of stars.
- Click here to find the original of Activity 1.
- w2 – The website of the European Space Agency offers an animation demonstrating what happens when an object gets too close to a black hole.
- w3 – The ESO website offers a video showing real data of stars orbiting a black hole.
- w4 – Hosted by Harvard University, the Inside Einstein’s Universe website offers a range of educational astronomy resources, including a downloadable demonstration manual and other materials about black holes.
- w5 – UNAWE is an astronomy programme to educate and inspire young childrenaround the world.
Resources
- The Hubblesite website from the Space Telescope Science Institute offers lots of information about black holes, as well as interactive, online activities and experiments.
- The Ask an Astronomer website at Cornell University offers accessible answers, aimed at different levels (beginner, intermediate, advanced), to many questions about black holes.
- The Kids Astronomy website provides a simple reference for young children to learn about black holes.
- To find out what happens when a massive star explodes in a supernova, see:
- Székely P, Benedekfi Ö (2007) Fusion in the Universe: when a giant star dies…. Science in School 6: 64-68.
- To learn more about the fusion reactions that occur in stars, and how light elements are fused into heavier ones, see:
- Boffin H, Pierce-Price D (2007) Fusion in the Universe: we are all stardust. Science in School 4: 61-63.
- Rebusco P, Boffin H & Pierce-Price D (2007) Fusion in the Universe: where your jewellery comes from. Science in School 5: 52-56.
- To complement this simulation of a black hole, you may like to model eclipses or the aurorae (northern and southern lights) in the classroom.
- Rosenberg M (2012) Creating eclipses in the classroom. Science in School 23: 20-24.
- Jeanjacquot P, Lilensten J (2013) Casting light on solar wind: simulating aurorae at school. Science in School 26: 32-37.
Review
In this article, the author briefly describes how black holes are formed in space and how they interact with what is known as ‘space–time’. She then describes very simple but impressive experiments to demonstrate the formation of black holes and how they can influence the space around them.
Suitable comprehension questions after the activities include:
- Describe black holes.
- What allows stars to be stable? (Your students could discuss gravity and fusion.)
- What is a singularity?
- How does gravity influence massive objects? What about photons (light)?
- What are supermassive black holes?
Gerd Vogt, HLUW Yspertal, Austria