Getting ahead in evolution Understand article
Lucy Patterson talks to Èlia Benito Gutierrez, from the European Molecular Biology Laboratory in Heidelberg, Germany, about how Èlia’s favourite animal, amphioxus, could be the key to understanding the evolution of vertebrates.
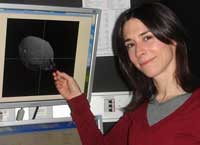
high-powered microscopes
to take a close look at the
developing amphioxus brain
Image courtesy of EMBL
Photolab
In coastal seabeds around the world, buried up to the gills, the worm-like amphioxus filters plankton from the waves. This has been going on for a very long time. Day in and day out, for more than 520 million years, amphioxus – or something very like it – has filter-fed as the world changed around it. Fish heaved themselves onto land, dinosaurs rumbled across the plains, early man struck flints together to make fire, and all the while, amphioxus sat there. If you have the chance to go snorkelling, look out for them. They might not sound very dynamic, but these creatures have fascinated natural historians and scientists since the mid-19th century, including Èlia Benito Gutierrez.
Her interest in amphioxus was sparked in her first-year zoology course at the Universitat de Barcelonaw1, Spain. “Amphioxus was this strange worm-like animal mentioned at the end of the list of invertebrates, before the vertebrates,” she remembers. Since Charles Darwin’s great revelation 150 years ago, many have considered amphioxus to be the key to understanding the origin of the vertebrates – the group of backboned animals including fish, amphibians, reptiles, birds and mammals, among them, of course, us. Èlia’s own niggling curiosity brought her, after a PhD in Barcelona and a research position in London, UK, to the European Molecular Biology Laboratory (EMBL)w2 in Heidelberg, Germany, and a pioneering new amphioxus project.
Èlia’s project falls under the still rather new science of ‘evo-devo’ – the combined study of evolution and development. Scientists are increasingly realising that the intricacies of development – how a single fertilised egg gives rise to the incredible diversity of cells and tissues in an adult – have had a major impact on the course of evolution.
Rather than re-inventing the wheel every time, new species arise through tweaks and adjustments to pre-existing developmental plans. The earlier the tweaks, the more dramatic the resulting changes. Later tweaks, producing subtler changes, were less likely to cause major disadvantages, and thus were favoured. This has mind-boggling implications: the further back in development we look, the more similar we are to our evolutionary ancestors. For instance, did you know that as embryos, we pass through a stage in which we start to develop gills like our fishy predecessors? Just like fish embryos, we develop ‘pharyngeal arches’, six fleshy pouches on either side of the neck, each containing a rod of cartilage. Evolutionary adaptations since our aquatic past caused these to be re-assimilated into the developing jaw and tiny bones of the middle ear.
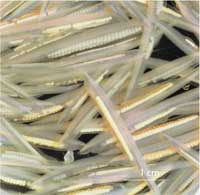
Image courtesy of Èlia Benito
Gutierrez
Amphioxus is one of a very select group of creatures that Darwin termed ‘living fossils’ – species alive today but still remarkably similar to their ancient fossilised ancestors – which are of great value to scientists studying evo-devo. Other examples include crocodiles and the coelacanth, a large ancient-looking fish thought to be extinct until a living specimen was discovered in 1938. Over millennia, Earth has witnessed huge environmental changes, stimulating the evolution of new species, and bringing about the extinction of others. In fact, it is calculated that 99.9% of all species that ever lived are now extinct.
Nonetheless, these fossil species have survived and appear relatively unchanged. Although it may seem that these creatures have been stuck in an evolutionary time warp, in fact their DNA has been subject to as many mutations as that of other species. However, for some reason, mutations that caused changes to body shape were never particularly advantageous. For amphioxus – a versatile creature that is comfortable in many kinds of sandy or gravelly seabed, in warm or even rather chilly water – perhaps the filter-feeding lifestyle it shares with its ancestors was, even over 520 million years, never really under threat.
However, what most excites amphioxus fans is the position of its ancient ancestor in the evolutionary tree. As Èlia explains, “what’s really interesting is that the ancient ancestor that amphioxus represents was a kind of minimalist or ‘stem’ vertebrate.” Although officially an invertebrate, amphioxus has a lot in common with backboned vertebrates. It has a hollow nerve cord running down its back, like our spinal cord, and next to this a notochord, a stiff but flexible rod that supports the body, serving as a kind of primitive backbone. As embryos, we also have a notochord, a remnant from our invertebrate past, but just like the pharyngeal arches, ours is broken up and re-used – to make the disks that lie between the vertebrae. “As the closest living relative of the ancestor of all vertebrates, amphioxus gives us a rare glimpse of how our evolutionary ancestors are likely to have looked,” Èlia says. And really, the chances of such an evolutionarily important fossil species being alive today must be phenomenally slim.
So how did the vertebrates evolve from these amphioxus-like ancestors? Of course, cartilage and bone evolution was very important, but the transition from invertebrate to vertebrate involved more than a backbone. It was also a matter of lifestyle choice, particularly feeding. Whereas creatures like amphioxus sat on the seabed, waiting for food to come to them, the early vertebrates evolved a new strategy: predation. They started to evolve the means to actively find food, requiring a whole raft of novel innovations, body parts and skills. Key to these was the evolution of a new head.
It might seem obvious, but to actively feed yourself, you first have to find your food. For this, you need sophisticated sensory organs to see, smell, taste and hear it (although our invertebrate ancestors had sensory cells or organs, the early vertebrates evolved paired organs, like our eyes, allowing them to sense the world in three dimensions). Where better to evolve these new, food-finding tools and the brain they’re wired up to than next to your mouth? These innovations, in turn, broadened the menu, and, for most vertebrates, the pursuit of less digestible diets resulted in the evolution of a jaw with teeth to bite and chew food before it reaches the gut.
Key to both the development and evolution of the head is a tissue called ‘neural crest’ – special cells originating from the same tissue that makes our brain and spinal cord. Once formed, these cells begin to migrate all over the body. The final destination of many is the head, where they make connective tissue, muscle, skin, facial nerves, bones and cartilage, providing support crucial to the development of the eyes and the taste and smell receptors of the mouth and nose. Neural crest cells also contribute (via the pharyngeal arches) to the jawbones, teeth, and the tiny bones of the middle ear, essential for the evolution of hearing.
As its name suggests (‘amphis’ means ‘both’ and ‘oxys’ means ‘sharp’; so ‘sharp at both ends’), amphioxus doesn’t have much of a head. Crucially, neither does it have neural crest, whereas all vertebrates do. There is some evidence though that migrating cells a little like primitive neural crest exist in amphioxus and in tunicates (sea squirts), another invertebrate group related to the ancestral vertebrate. By studying these cells, Èlia hopes to understand how neural crest, and by extension, the head, evolved.
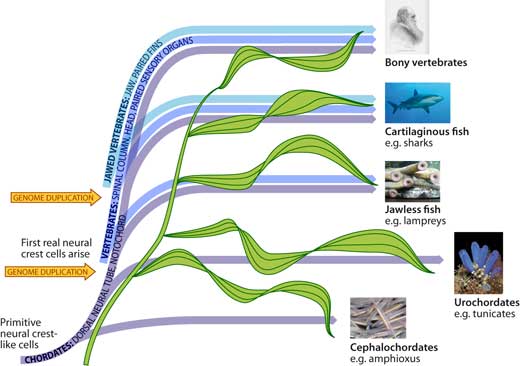
However, in 2006, by looking at DNA, scientists discovered that, in fact, vertebrates share a more recent common ancestor with tunicates than with amphioxus. This common ancestor would probably also have looked similar to amphioxus, but since our lineages split, our evolution has taken a very different path: vertebrates evolved neural crest, a head and predation, while the tunicates specialised even further to become (as adults) sedentary filter feeders
Image courtesy of Lucy Patterson. Constituent images courtesy of Lycaon (amphioxus), Nhobgood (sea squirt), Drow_male (lampreys), Albert Kok (shark), Robbot (Darwin), Lmozero (hagfish); image source: Wikimedia Commons
Since the completion of the amphioxus genome sequence in 2008, amphioxus research has really come of age. Scientists like Èlia can now work out how the stem vertebrate genome would have looked. Comparisons with vertebrate genomes, including human, show remarkable similarity. “We now know that amphioxus has all the same important gene families that vertebrates have,” explains Èlia. “All the basic building blocks needed to make a vertebrate are present. So you could ask: why doesn’t amphioxus develop like a vertebrate?” The likely answer to this also lies in the genome. Unlike in vertebrates, where many genes are duplicated, all amphioxus gene families are present as single copies. This confirms suspicions of a major difference between vertebrates and their ancient ancestor: early in the evolution of vertebrates, the entire ancestral genome was duplicated, twice.
Strange events in our evolutionary history at once vastly increased the number of genes available for natural selection to act on, and therefore the potential to evolve. With the original genes taking care of the usual business of building an animal, there was much greater freedom for natural selection to play around with the new copies. Through this activity, whole families and networks of genes were recycled to make new body parts and systems. Indeed, it’s only after the genome duplications that neural crest evolution really took off and vertebrates started to acquire a head. Quite when and how these duplications occurred is unclear and, over millions of years of subsequent evolution, most of the copied genes – those that didn’t give rise to new features – have been lost.
So, rather than just sitting in the sand, with no real pressure to change, perhaps amphioxus has simply evolved as far as it could with the genes it has; without the influx of freshly copied genes, it has reached a dead end. Whereas for many other species, such a dead end would mean extinction, there is always a part of the coastline somewhere where amphioxus can bury itself.
Researchers like Èlia are more than happy that amphioxus is still around today because it provides a unique opportunity to study how the early vertebrates evolved. Her particular interest is in the evolution of the brain, and she plans to construct a detailed map of the amphioxus brain as it develops, showing the positions of the different neurons and which genes they express. “By comparing amphioxus to vertebrate species we can learn how the basic machinery already present in the stem vertebrate was recruited and redeployed, eventually creating complex characteristics like memory or our ability to learn.” So by getting left behind for all those years, amphioxus might provide the key to our evolutionary past, helping us to understand the creatures we are today.
Web References
- w1 – For more information on the Universitat de Barcelona, see: www.ub.edu
- w2 – Find out more about the European Molecular Biology Laboratory here: http://www.embl.org
Resources
- To read more about the research group at EMBL where Èlia works and the story of her supervisor, Detlev Arendt, see:
- Hodge R (2006) A search for the origins of the brain. Science in School 2: 68-71. www.scienceinschool.org/2006/issue2/brain
- To learn about how a new tree of life – tracing the course of evolution – was established, see:
- Hodge R (2006) A new tree of life. Science in School 2: 17-19. www.scienceinschool.org/2006/issue2/tree
- The Understanding Evolution website from the University of California, Berkeley (USA), provides authoritative, up-to-date information about evolutionary mechanisms, theory, evidence and modern research. The site includes numerous resources for teaching about evolution, including evo-devo (aimed at a US audience). See: Berkeley’s evolution website (http://evolution.berkeley.edu) or use the direct link: http://tinyurl.com/yc27f4n
- A great feature article on evo-devo from The New York Times:
- Yoon CK (2007) From a Few Genes, Life’s Myriad Shapes. The New York Times 26 June. See the New York Times website (www.nytimes.com) or use the direct link: http://tinyurl.com/34h675
- Two popular science books about evo-devo research:
- Shubin N (2008) Your Inner Fish. A Journey into the 3.5 Billion-Year History of the Human Body. London, UK: Allen Lane. ISBN: 9780713999358
- Carroll SB (2005) Endless Forms Most Beautiful: The New Science of Evo Devo and the Making of the Animal Kingdom. New York, NY, USA: Norton. ISBN: 9780393060164
- A comment on the importance of the publication of the amphioxus genome, including some more information about amphioxus, from Nature:
- Gee H (2008) Evolutionary biology: The amphioxus unleashed. Nature 453: 999-1000. doi: 10.1038/453999a. Download the article free of charge here, or subscribe to Nature today: www.nature.com/subscribe
- The amphioxus song: www.molecularevolution.org/mbl/resources/amphioxus
- A video of a coelacanth: www.youtube.com/watch?v=NzzxOlFJtzg
- Watch this incredible talk from paleontologist Paul Sereno. In just over 20 minutes he explains his research – digging for fossils in brand new territories – how his encounters with living crocodiles have revealed how huge ancient fossilised crocodiles would have looked, and how he hopes this kind of research will help to inspire future generations of scientists. See: www.ted.com/talks/view/id/428
- Read more about natural selection and molecular evolution in:
- Bryk J (2010) Natural selection at the molecular level. Science in School 14: 58-62. www.scienceinschool.org/2010/issue14/evolution
- For a review of a book on evolution that is suitable for beginners, see:
- Haubold B (2010) Review of Why Evolution is True. Science in School 14. www.scienceinschool.org/2010/issue14/evotrue
Institutions
Review
This fascinating article emphasises the importance of the science of evo-devo and how the invertebrate amphioxus has evolutionary links with vertebrates, ranging from the development of the head to an explanation for a slipped disc. The breadth of information covered in the article makes it an invaluable resource for teachers and for students aged 16-18.
The information could be used for teaching vertebrate evolution, in particular the development of the nervous system and sensory organs, and for group discussion to address why amphioxus provides a valuable model for vertebrate development. There are interdisciplinary opportunities with geology (the fossil record), and with information and communications technology (the construction of phylogenetic trees).
The article would make an excellent comprehension exercise, using, for example, the following questions.
- Why is evo-devo an important science?
- What is meant by a living fossil?
- What do you understand by the terms ‘stem vertebrate’ and ‘stem genome’?
- What were the selection pressures that led to the development of the head?
- What do you understand by the term ‘gene duplication’ and why was it crucial to vertebrate development?
For the more adventurous, there is the potential for DNA sequence alignment and phylogenetic analyses between amphioxus and human genes.
Mary Brenan, UK
Referee’s recommendations: Biology, Earth sciences, Information and communications technology, Evolution, Development, Geology
Mary Brenan, UK