The first light in the Universe Understand article
Ana Lopes and Henri Boffin take us on a trip back in time – probing the history of the Universe.
Have you ever wondered when the first lights in the Universe began to shine? Most of us have watched the rising of the Sun in the morning, the dawn of a new day. Astronomers go a step further and look for the first sources of light – peering into the history of the Universe using powerful telescopes. Their ultimate aspiration is even more ambitious: to trace the entire history of the Universe, from its birth – the Big Bang – to the present day, almost 14 000 million (14 billion) years later.
Photographs of the Universe
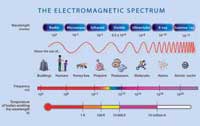
spectrum. Click to enlarge
image
Image courtesy of NASA
It was not until about 400 000 years after the Big Bang that light was able to travel freely in the Universe. Ever since the Big Bang, the Universe has been expanding and cooling (for a description, see Boffin & Pierce-Price, 2007), stretching that primeval light from its initial high frequency, until it can be detected today as photons in the microwave range: cosmic microwave background radiation, coming from all over the Universe.
Historians often use photographs and other pictures to tell them about the past, and in this respect, astronomers are no different.
Using the COBE (COsmic Background Explorer) and Wilkinson Microwave Anisotropy Probe (WMAP)w1 satellites to map the cosmic microwave background radiation, astronomers have created a ‘photograph’ of the Universe as it was approximately 400 000 years after the Big Bang. The data from COBE won John Mather and George Smoot the 2006 Nobel Prize in Physics.w2
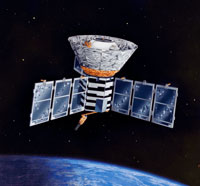
COBE spacecraft, launched by
NASA into an Earth orbit in
1989 to make a full-sky map
of the cosmic microwave
background radiation left
over from the Big Bang. The
first results were released in
1992
Image courtesy of NASA /
COBE Science Team
The standard cosmological model of how the Universe evolved tells us that by about 400 000 years after the Big Bang, the Universe had cooled to about 3000 degrees Kelvin, a temperature low enough for all the electrons and protons to combine, forming neutral hydrogen from the ionised gas. Electrons in neutral hydrogen (as in other atoms or molecules) absorb photons very efficiently, so a universe full of neutral hydrogen is opaque. In contrast, when protons and electrons are separate, they cannot capture photons so a universe full of ionised gas – as was the case until about 400 000 years after the Big Bang, and is again the case today – is relatively transparent. The COBE and WMAP maps show us the Universe during its opaque stage, at the beginning of the ‘dark ages’ of the Universe. This period came to an end when the Universe became ionised again (see diagram on the right).
We also have ‘photographs’ of a much more recent Universe: galaxies full of stars, as they were 1000 million years after the Big Bang – once the Universe had become transparent again. Because of the finite speed of light (300 000 km s-1), the light from distant objects takes much longer to reach us than that from neighbouring objects; we therefore see these distant objects as they were a very long time ago. By looking at very distant objects, astronomers were able to see the light that has travelled for almost 13 000 million years – that is, they saw those objects as they had been less than 1000 million years after the Big Bang.
But what happened between these two photographs, between the release of the cosmic microwave background radiation 400 000 years after the Big Bang and the light emitted by these very distant galaxies, nearly 1000 million years later? When and how did the cosmic fog lift? What changed a sea of particles almost devoid of structure into a Universe lit by numerous stars in young galaxies?
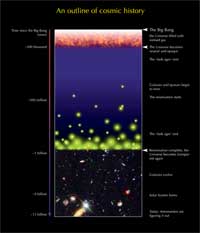
Click to enlarge image
Original image courtesy of SG
Djorgovski and Digital Media
Center, Caltech
As Harvard University astronomer Abraham Loeb puts it: “The situation that astronomers face is similar to having a photo album containing the first ultrasound image of an unborn baby and some additional photos of that same person as a teenager and an adult” (Loeb, 2006). What scientists do not know – but are trying to figure out – is when and how the very first stars and galaxies were born. Loeb continues: “Astronomers are currently searching for the missing pages of the cosmic photo album, which will show how the Universe evolved during its infancy and made the building blocks of galaxies like our own Milky Way.”
Before any stars were formed, the Universe contained mostly hydrogen, helium and some traces of light elements (as described in Rebusco et al., 2007). To ionise hydrogen requires energy in excess of 13.6 eV – the sort of energy level corresponding to photons in the ultraviolet (UV) domain. Therefore whatever reionised the Universe must have released significant amounts of UV radiation.
Although astronomers are still uncertain what could have released this ionising UV radiation, they speculate that it was either the first, very hot, stars, or early black holes, releasing huge amounts of UV radiation as material fell into them. If this is the case, stars must have formed before the reionisation epoch – thus if we can date the reionisation, we have at least a latest date for the emergence of the first stars.
The ultraviolet footprint
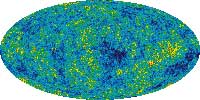
of the infant universe created
from five years of WMAP
data. The image reveals 13.7
billion-year-old temperature
fluctuations (red regions are
warmer and blue are cooler)
that correspond to the seeds
that grew to become the
galaxies
Image courtesy of NASA /
WMAP Science Team
In 1965, American astronomers James Gunn and Bruce Peterson predicted that the spectra of quasars could be used to date the final stages of the reionisation epoch. Quasars are very distant and ancient galaxies of extreme brilliance, thought to be powered by material falling into giant black holes at their centres. If the quasar is so distant that the light we observe from it escaped during the ‘dark ages’, its UV light will have been absorbed by the neutral hydrogen present at the time; if the quasar is closer and the light we observe was emitted only after the reionisation, there will have been no neutral hydrogen to impede it (see diagram below). (Note that while the neutral hydrogen atoms absorb all wavelengths of light, most wavelengths are released again. UV light, in contrast, ionises the atoms and is completely absorbed.)
If even a tiny portion of the intergalactic medium (as little as one part in a million) had been neutral when the quasar released the light we now see from Earth, this would have left a noticeable imprint on the spectrum – a suppression of light in the UV range, known as the Gunn-Peterson trough.
Therefore James Gunn and Bruce Peterson predicted that quasars beyond a certain distance from Earth, for which we observe light that was released before the reionisation had finished, would show a ‘trough’ in their spectra. Quasars closer than this would not – they released the light we observe from Earth only after the reionisation had finished.
In 2001, a team of scientists led by Robert Becker from the University of California, USA, confirmed Gunn and Peterson’s prediction: they detected an unambiguous trough in the spectrum of a very distant quasar discovered during the Sloan Digital Sky Surveyw3, a huge astronomical survey that examined the spectra of about a hundred thousand quasars. The trough was in the infra-red part of the spectrum, because the quasar is so far away: its light started the journey towards Earth only about 900 million years after the Big Bang, and so has taken almost 13 000 million years to reach us, during which time its initially UV light has been stretched (redshifted) into the infra-red by the expansion of the Universe. Quasars slightly closer to Earth did not show such a trough. This indicated that the last patches of neutral hydrogen in the Universe were ionised about 900 million years after the Big Bang.
The microwave footprint
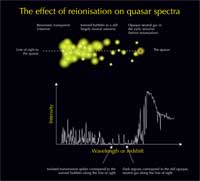
quasar spectra. Click to
enlarge image
Image courtesy of SG
Djorgovski and Digital Media
Center, Caltech
The cosmic microwave background radiation released soon after the Big Bang is another source of information about the reionisation epoch.
As the Universe started to be reionised, the electrons that were released affected the polarity of light. A free electron can interact with a photon in a process called Thomson scattering: the electron is accelerated and the incident light is polarised along the direction of motion of the electron. This effect was most pronounced during and just after the reionisation; later, because the Universe continued to expand, the density of free electrons decreased, reducing their polarising effect.
Between 2001 and 2006, the WMAP satellitew1 was used to study the degree of polarisation of the cosmic microwave background photons. By looking at different frequencies of light, astronomers could look at different periods in the Universe’s history – and the degree of polarity gave an indication of the density of free electrons around at that time (the greater the polarity, the higher the density of free electrons). From these studies, they concluded that reionisation started about 400 million years after the Big Bang, and was completed 400 to 500 million years later. This is in agreement with the findings of the quasar studies: 900 million years after the Big Bang.
Future research
On 14 May 2009, the European Space Agencyw4 launched the Planck satellitew5 to provide us with a photograph of the cosmic background radiation with even more sensitivity and angular resolution than the WMAP achieved. It will certainly help astronomers to answer with more details the questions of how the Universe evolved from a glowing soup to what we see today.
Although the time when reionisation occurred has been successfully identified, a photograph of the Universe from that time is still lacking, as current telescopes are unable to image it. The good news, however, is that the European Southern Observatory (ESO), together with astronomers and engineers from across Europe, is now engaged in the design of the European Extremely Large Telescopew6, 42 m in diameter, which will allow us to look this far back in time and possibly see the first starlight.
References
- Boffin H, Pierce-Price D (2007) Fusion in the Universe: we are all stardust. Science in School 4: 61-63.
- Loeb A (2006) The dark ages of the Universe. Scientific American Nov: 46-53. This article is available to download from www.cfa.harvard.edu/~loeb/sciam.pdf
- Rebusco P, Boffin H, Pierce-Price D (2007) Fusion in the Universe: where your jewellery comes from. Science in School 5: 52-56.
Web References
- w1 – For more information about the WMAP satellite, see: http://map.gsfc.nasa.gov
- w2 – An overview of John Mather and George Smoot’s work on cosmic microwave background radiation with COBE and links to further information are given in the press release announcing their Nobel Prize: http://nobelprize.org/nobel_prizes/physics/laureates/2006/press.html
- w3 – The Sloan Digital Sky Survey is the most ambitious astronomical survey ever undertaken. When completed, it will provide detailed optical images covering more than a quarter of the sky, and a three-dimensional map of about a million galaxies and quasars. As the survey progresses, the data are released to the scientific community and the general public in annual increments. See: www.sdss.org
- w4 – For more information about the European Space Agency, see: www.esa.int
- w5 – To learn more about the Planck satellite, see: www.esa.int/esaSC/120398_index_0_m.html
- w6 – For more information about ESO’s Extremely Large Telescope, see: www.eso.org/public/astronomy/teles-instr/e-elt.html
Resources
- The WMAP section of the NASA website provides some teacher resources, including a brief overview of the WMAP project and an inflatable model of the Universe. See: http://map.gsfc.nasa.gov/resources/edresources1.html
- You may also enjoy the following articles:
- Larson RB, Bromm V (2001)The first stars in the Universe. Scientific American Dec: 64-71. This article is available for download from www.astro.yale.edu/larson/papers/SciAm01.pdf
- Madau P (2006) Astronomy: trouble at first light. Nature 440: 1002-1003. doi:10.1038/4401002a. Download the article free of charge here, or subscribe to Nature today: www.nature.com/subscribe
- Scannapieco E, Petitjean P, Broadhurst T (2002) The emptiest places. Scientific American Oct: 56-63. This article is available to download from http://scannapieco.asu.edu/papers/sciam.pdf
Institutions
Review
This article offers interesting and detailed information about modern research into the history of the Universe and its evolution. It could be used for interdisciplinary teaching, for example in physics, astronomy, astrophysics or philosophy. As well as providing valuable background reading, teachers could use it to develop educational materials.
Vangelis Koltsakis, Greece