More than meets the eye: the cold and the distant Universe Understand article
In the fifth and final article in this series on astronomy and the electromagnetic spectrum, find out how scientists use the European Space Agency’s missions to observe the sky in far-infrared, sub-millimetre and microwave light.
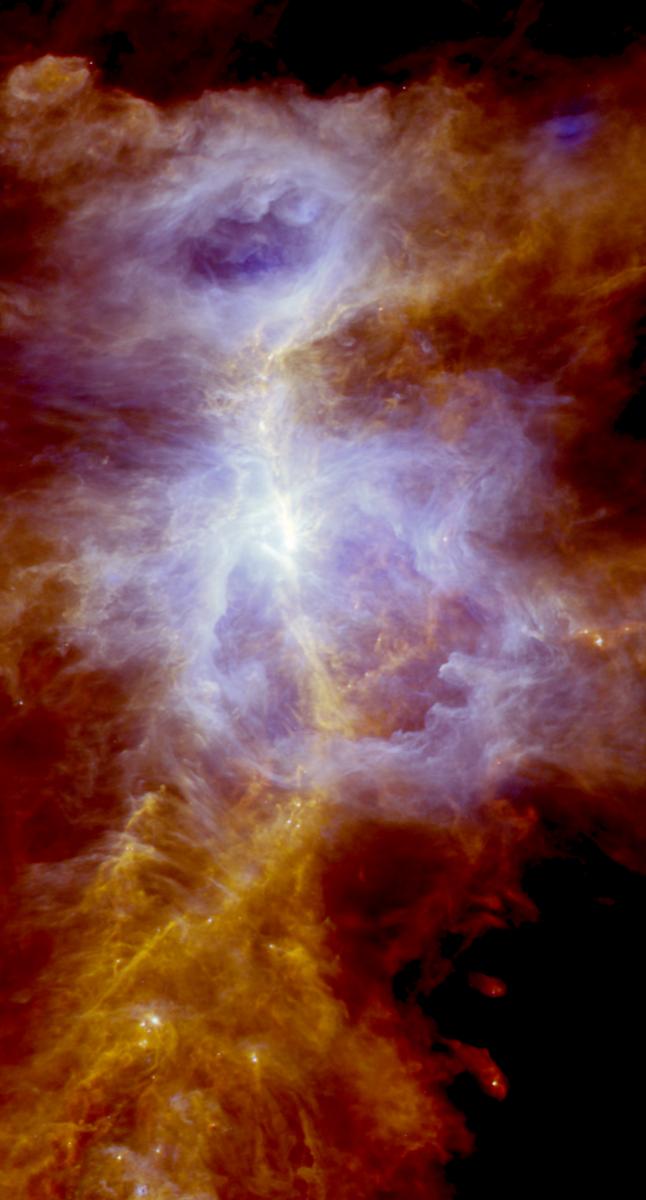
cloud seen by ESA’s Herschel
Space Observatory
Image courtesy of ESA /
Herschel / Ph André, D
Polychroni, A Roy, V Könyves,
N Schneider for the Gould Belt
survey Key Programme
Five thousand light years from Earth lies the coldest object found in the Universe, the Boomerang Nebula – a dying star leaving behind a cloud of gas that is only one degree above 0 K – absolute zero. This cloud, like other cold objects in the Universe, is invisible to the naked eye.
The cooler an object is, the longer the wavelengths of light it emitsw1. With temperatures of 50 K or less, cool portions of interstellar gas and cosmic dust emit light at far-infrared (25 to 350 μm) and sub-millimetre (350 μm to 1 mm) wavelengths, much longer than our eyes can see. So how do we know that these cold objects exist? To capture the radiation and ‘see’ the objects in wavelengths beyond the visible range, astronomers use dedicated far-infrared (FIR), sub-millimetre (sub-mm) and microwave telescopes.
This approach comes with challenges: light at these long wavelengths is absorbed by water vapour and other molecules in Earth’s atmosphere, which makes observations from the ground extremely difficult and, at FIR wavelengths, simply impossible. For most infrared wavelengths, the atmosphere itself also emits light, adding an unwanted source of noise to the cosmic signals that astronomers are interested in.
To combat these problems, long-wavelength telescopes can be located in dry, high-altitude regions. The world’s largest radio astronomy facility – the Atacama Large Millimeter/submillimeter Array (ALMA) – is in the Chilean Andes, for example. At an altitude of 5000 metres, ALMA is one of the highest observatory sites on Earth, studying light from some of the coldest objects in the Universe (as described in Mignone & Pierce-Price, 2010).
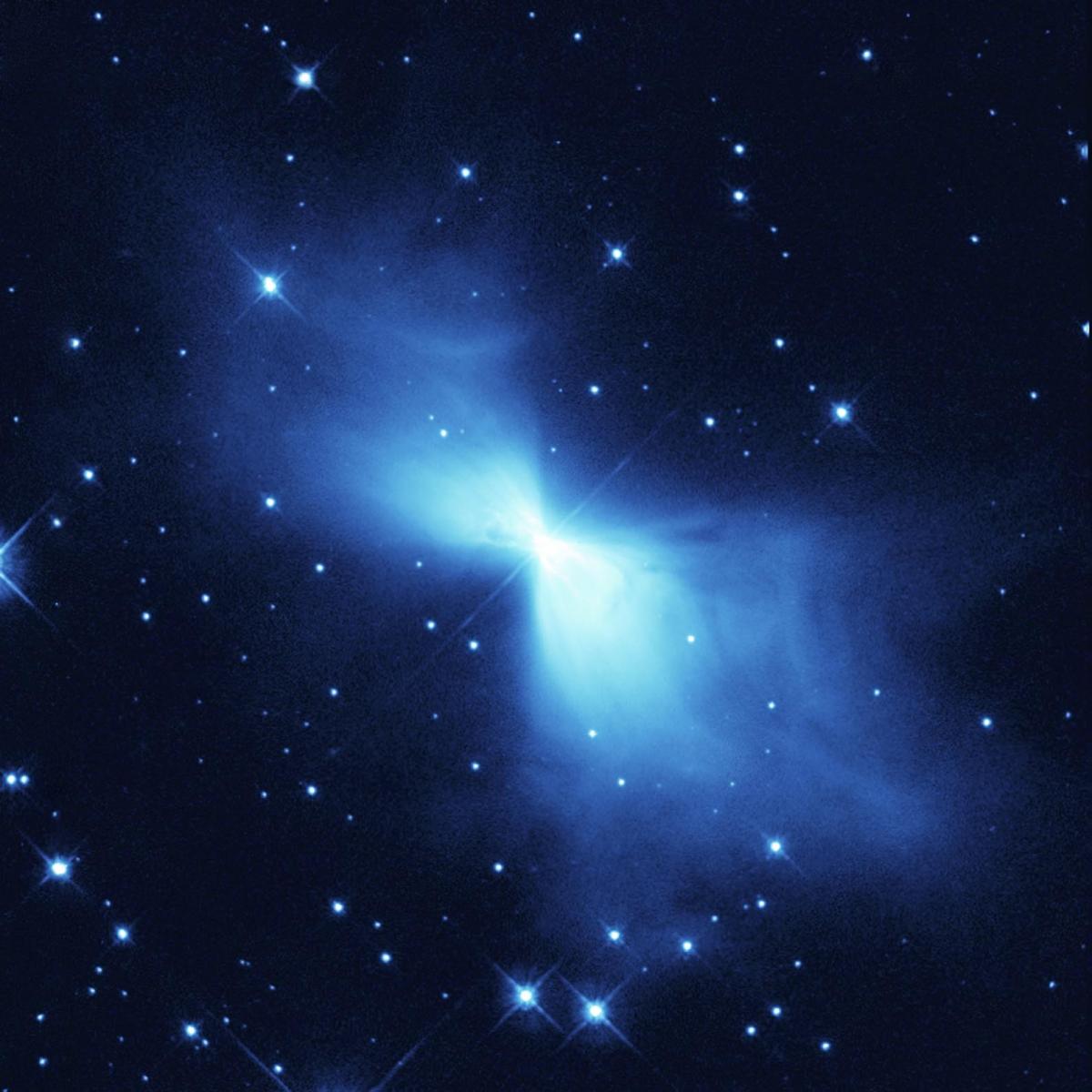
young planetary nebula and
the coldest object found in
the Universe so far.
Image courtesy of ESA / NASA
The European Space Agencyw2 (ESA) journeyed even higher on 14 May 2009 when they launched two new space observatories. Operating beyond Earth’s atmosphere, the Herschel Space Observatory and the Planck satellite studied the cold and the distant Universe. In astronomical terms, looking at distant objects means looking back in time. When a telescope observes a galaxy 100 million light years away, we see the galaxy as it was 100 million years ago when the light was emitted. And because our Universe is expanding, the wavelength of light emitted by distant stars and galaxies is stretched even longer by the time it reaches telescopes on or near Earth – a phenomenon known as redshiftw3.
The Herschel Space Observatory comprised a 3.5 m telescope for FIR and sub-mm observations, and its mission was to study the origin and evolution of stars and galaxies. The Planck satellite’s goal, on the other hand, was to study the relic radiation from the Big Bang by surveying the entire sky in sub-mm and microwave wavelengths. Until 2013, when the two missions ended, their observations provided many missing clues for astronomers.
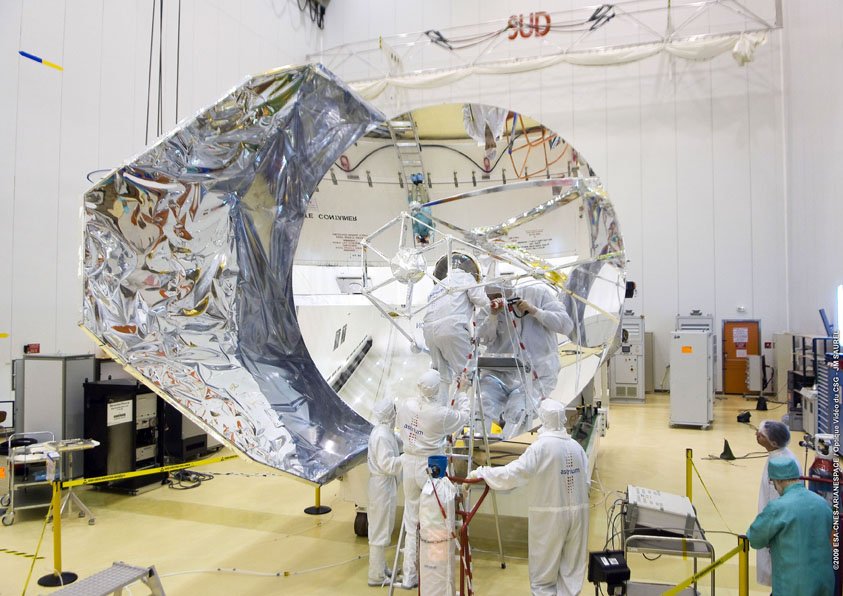
Image courtesy of ESA-CNES-Arianespace / Optique Vidéo du CSG
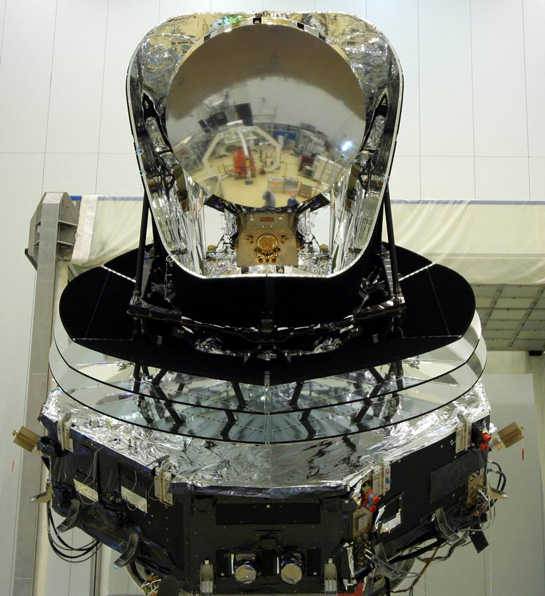
Image courtesy of ESA / Thales
How stars are born
What stands out in observations from long-wave telescopes is the cold mixture of gas and dust that pervades galaxies. This interstellar medium is the raw material from which stars and planets are born: within the densest parts of molecular clouds, gravity causes the gas and dust to contract and fragment, eventually leading to stellar birth.
While fully fledged stars shine most brightly in ultraviolet, visible and near-infrared light (as described in Mignone & Barnes, 2014), the earliest stages in star formation are best revealed in other portions of the electromagnetic spectrum. In particular, individual proto-stars within the Milky Way and in nearby galaxies can be detected at FIR and sub-mm wavelengths.
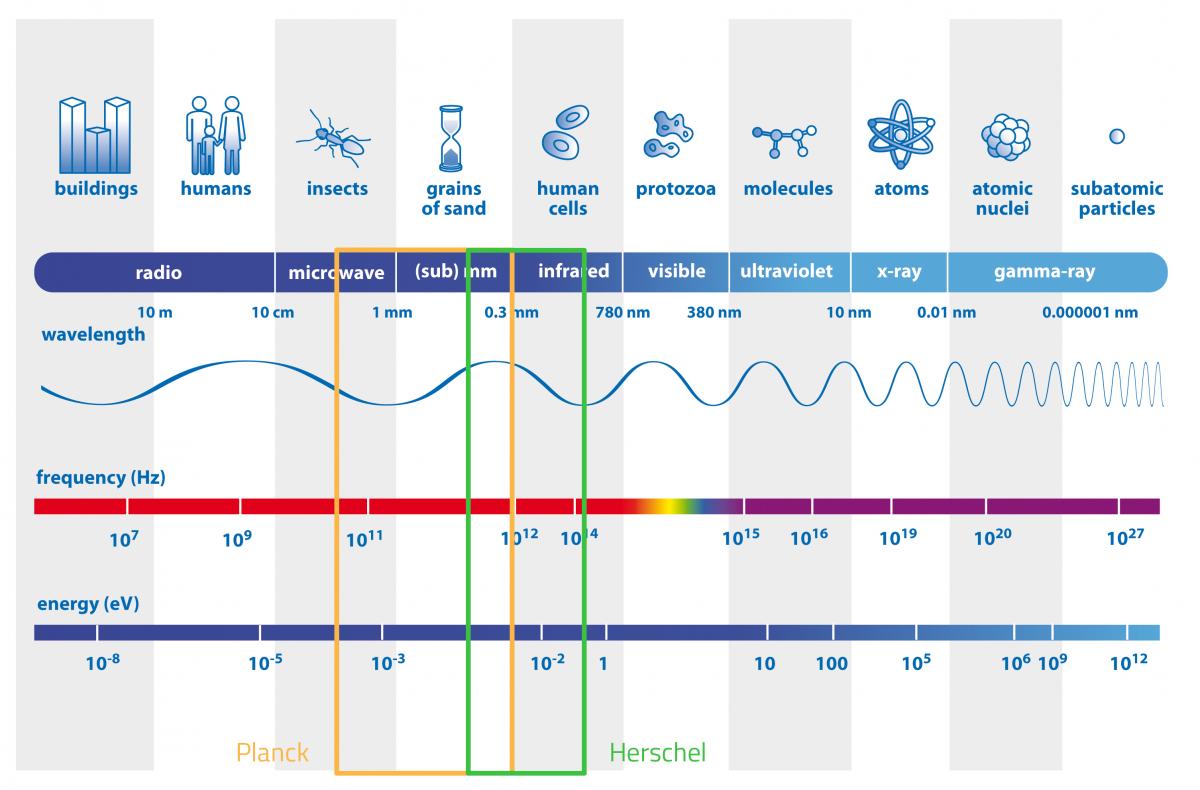
Image courtesy of ESA / AOES Medialab
Observations from the Herschel telescope revealed that the interstellar medium in our galaxy is threaded with filamentary structures of gas and dust on every scale. From nearby clouds hosting tangles of filaments a few light years long to gigantic structures stretching hundreds of light years across the Milky Way’s spiral arms, these structures – only a few of which were known prior to the Herschel mission – appear to be everywhere.
Astronomers now believe that filaments are key to star formation: once the density of interstellar gas and dust in a filament exceeds a critical value, it can become gravitationally unstable, giving rise to denser concentrations of matter that might eventually form stars.
Scanning the entire sky, the Planck satellite detected thousands of cold and dense clumps where stars are born and showed that these clumps are not isolated but appear to be all linked to one other. They form huge filamentary structures across our Milky Way, resembling the smaller filaments detected by the Herschel Space Observatory.
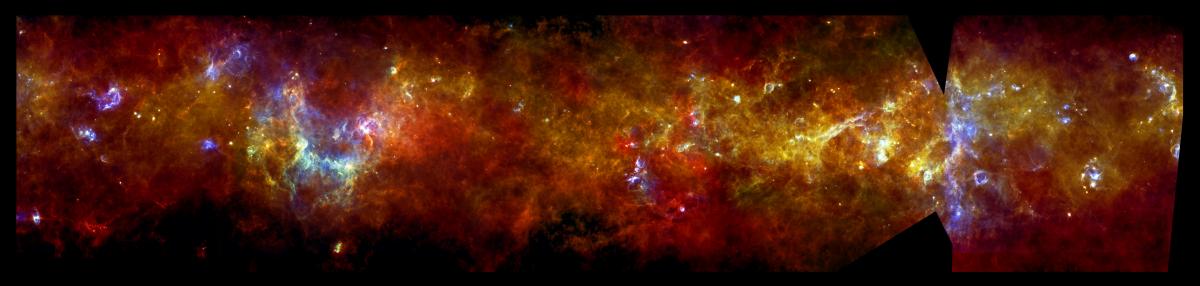
Image courtesy of ESA / PACS & SPIRE Consortium / S Molinari, Hi-GAL Project
The formation and evolution of galaxies
Observing star-forming regions of the Milky Way provides a window into the processes that give birth to stars closer to Earth now. However, the Herschel mission was also instrumental in investigating the evolution of star formation in galaxies throughout the history of the cosmos.
For example, studies based on Herschel observations have indicated that most stars in the history of the Universe have formed quietly in galaxies that are considered ‘normal’ for the epoch in which we see them, rather than through violent and tumultuous events such as the mergers of galaxies.
Mergers, although spectacular, are relatively rare and of short duration. They have not dominated the cosmic history of star formation for at least the past 10 billion (1010) years. What is crucial for star formation is that galaxies have sufficient gas available to create stars, which could be provided by intergalactic streams of cold gas.
The early Universe
Ultimately, the oldest light in the history of our 13.8 billion-year-old Universe is the cosmic microwave background (CMB) – the remains of thermal radiation from the Big Bang. A fossil from the hot and dense state of the early cosmos, the CMB was released only 380 000 years after the Big Bang and is the furthest back in time that we can explore using light. It contains a wealth of information about the formation and evolution of structure in the Universe and can be detected using microwaves.
Planck was the third space mission to survey this relic of the early Universe over the entire sky, after NASA’s COBE and WMAP satellites. In unprecedented detail, the Planck satellite mapped the tiny differences in the temperature of the CMB – a mere 0.00001 K plus or minus the background temperature of 2.73 Kw4.
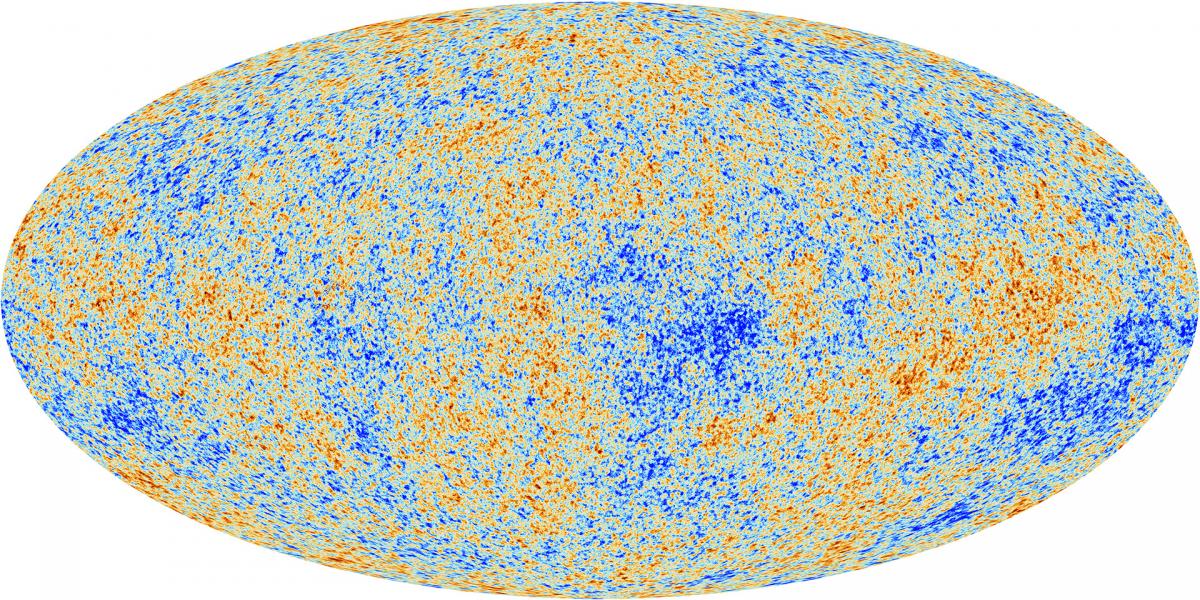
Image courtesy of ESA / Planck Collaboration
These minuscule fluctuations trace regions of slightly different density in the fluid that filled the early cosmos, before any stars or galaxies had formed. As such, they are the seeds around which all future cosmic structures, including the stars and galaxies of today, would later take shape.
Planck’s map is the most precise picture of the early Universe so far, confirming the standard view of the cosmos and allowing astronomers to estimate its age, expansion rate and composition with even greater accuracy.
Web References
- w1 – Find out how the wavelength at which a celestial object emits most of its light is related to the object’s temperature.
- w2 – ESA is Europe’s gateway to space, with its headquarters in Paris, France.
- w3 – Read more about redshift and its importance in astronomy.
- w4 – Learn more about the Planck satellite and the cosmic microwave background.
Resources
- To learn more about ESA’s Planck and Herschel missions, watch episodes two and three of the Science@ESA vodcasts.
- Herschel and Plank were equipped with state-of-the-art refrigeration systems to keep the detectors a few degrees above absolute zero.
- Explore the Online Showcase of Herschel Images.
- For more freely available education materials produced by ESA, see: www.esa.int/educationmaterials
- To read previous articles in the EM astronomy series, see:
- Mignone C, Barnes R (2011) More than meets the eye: the electromagnetic spectrum. Science in School 20: 51–59.
- Mignone C, Barnes R (2011) More than meets the eye: unravelling the cosmos at the highest energies. Science in School 21: 57–64.
- Mignone C, Barnes R (2012) More than meets the eye: the exotic, high-energy Universe. Science in School 24: 53–58.
- Mignone C, Barnes R (2014) More than meets the eye: how space telescopes see beyond the rainbow. Science in School 29: 49–54.
- Read about ALMA, the world’s largest radio astronomy facility. See:
- Mignone C, Pierce-Price D (2010) The ALMA Observatory: the sky is only one step away. Science in School 15: 44–49.
Institutions
Review
This article demonstrates how astronomers begin to answer the most probing of questions about the origins of the Universe and how stars formed in the early (and aged) Universe.
It could be used to develop discussions based on questions such as:
- How do astronomers use the electromagnetic spectrum?
- What can be gained from studying the cosmic microwave background (CMB)?
- Can the cost of scientific space missions be justified?
Robert Woodman, Ysgol Bro Gwaun, UK