Towards a better lithium-ion battery Understand article
Watching what happens to the electrodes in a lithium-ion battery with neutron science.
Lithium-ion batteries power our phones and laptops. They are even starting to power our cars, but the development of electric cars has been hindered by slow progress in battery technology. To help speed up this process and improve lithium-ion battery technology even more, my colleagues and I at Institut Laue-Langevin (ILL)w1 recently made a supersized battery so that they could see what happens inside while it is operating. The resulting ‘movie’ helped the scientists to see how they could improve the electrodes in the battery.
The power of lithium
We know lithium as the reactive group-one metal in the top left-hand corner of the periodic table. One of only three elements created in the Big Bang, lithium doesn’t just power your laptop – it is also burned up as star fuel.
Back on Earth, we get our lithium from the ground, where huge underground lakes containing lithium salts are tapped to generate about 25 000 tonnes of lithium metal every year. Much of this is used to make lightweight, rechargeable lithium-ion batteries.
Like all batteries, lithium-ion batteries store electrical energy using chemical potentials. Unlike other batteries, lithium-ion batteries are rechargeable so its reactants can be generated again just by passing electricity through the products. Once the battery has gone flat, it can be connected to a recharger which uses electrical energy to reverse the chemical reactions that took place in the battery while it was in use.
Unsurprisingly, lithium-ion batteries work by using positively charged lithium ions to transfer charge. Like all batteries, the battery is made up of two electrodes: one positive and made from a compound containing lithium (often with a transition metal oxide, for example manganese); and one negative, often carbon. These two electrodes are connected by an electrolyte that allows the charge carriers (lithium ions) to move between the two electrodes. The two half reactions are, for example:
LiMn2O4 ⇌ Li1-xMn2O4 + xLi+ + xe–
xLi+ + xe– + xC6 ⇌ xLiC6
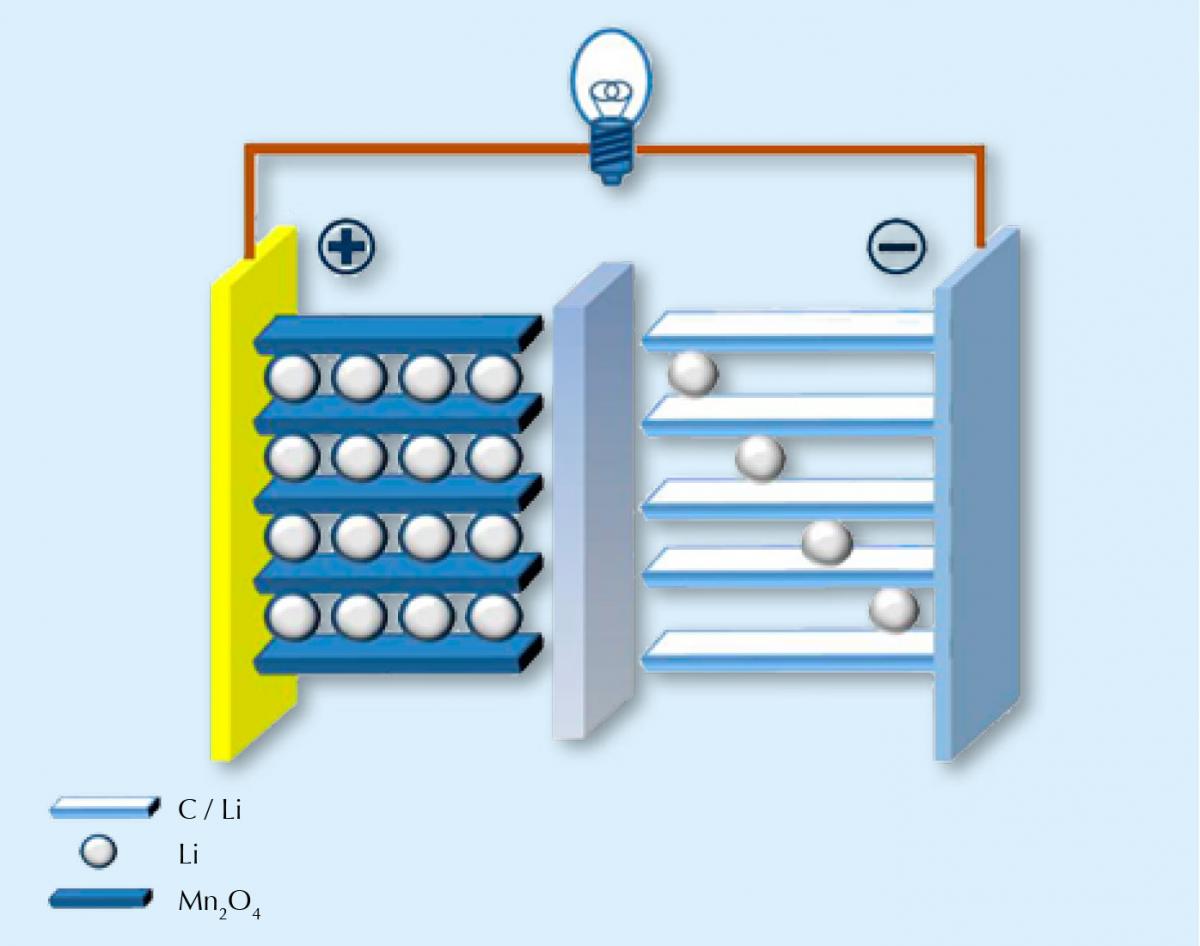
Image in the public domain by Chem511grpThinLiBat; image source: Wikimedia Commons
When the battery is discharging (i.e. when it is supplying power), the lithium ions move from the carbon electrode back to the lithium-containing electrode and then back in the opposite direction when the battery is recharged. Unfortunately, this backwards and forwards process can’t go on forever. Over time, lithium ions passing in and out of the electrodes cause them to swell and shrink repeatedly, thereby degrading them slowly. Much like how your favourite clothes lose their fit and fray around the edges as you repeatedly wear and wash them. Eventually your favourite jeans don’t fit you as well as they used to, and in a similar way, the lithium ions can’t fit into the lattice of the electrodes so well. The battery loses capacity.
To improve batteries, therefore, you need to improve the electrode materials – which means you need to understand how these materials change with repeated charge and discharge cycles. This is where ILL and its neutron source come in, allowing us to ‘visualise’ the changes in the crystal structure of the electrodes. Neutron diffraction is an excellent technique for seeing lithium ions moving through the electrodes because neutrons are readily scattered by light elements such as lithium.
Real-time movies
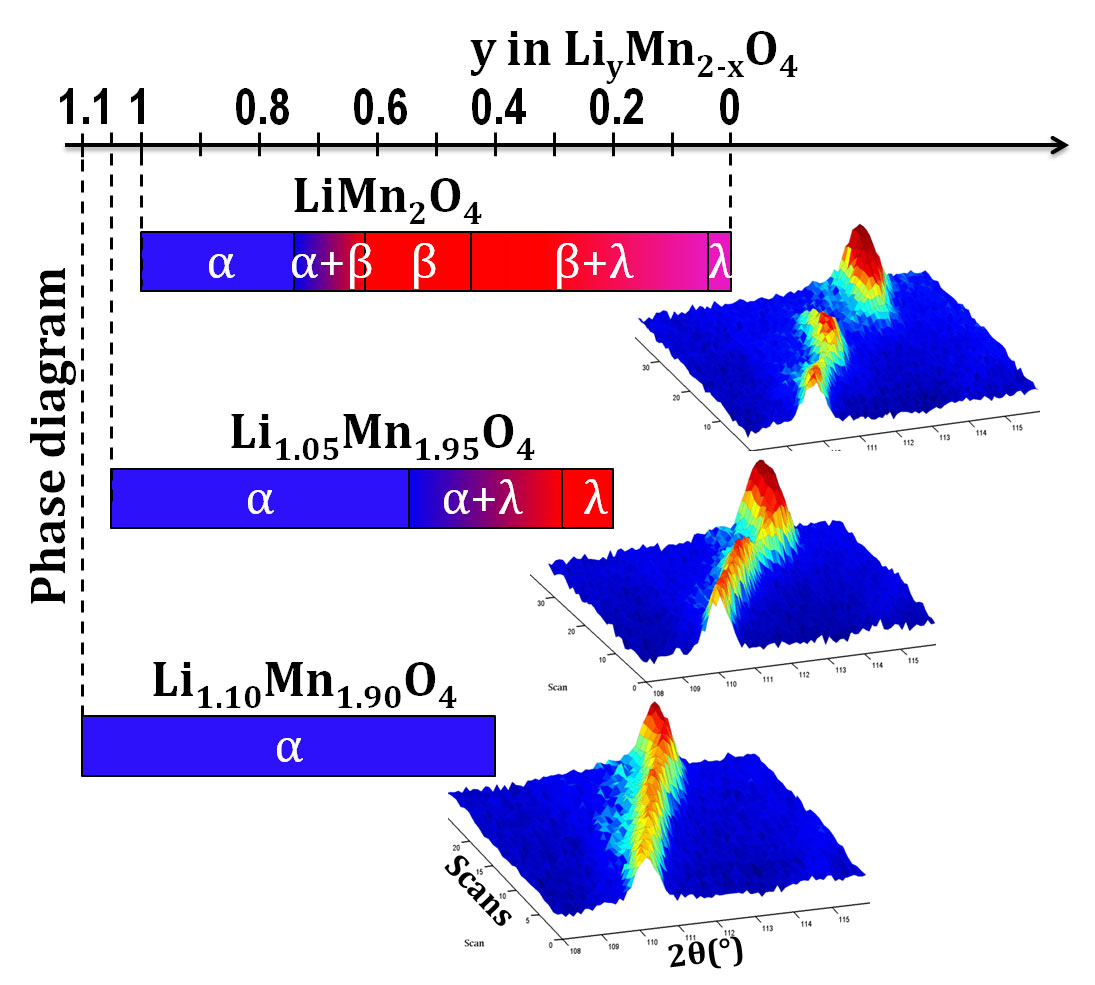
electrodes undergo different
changes as they are charged
and discharged
Image courtesy of the author
For this reason, we developed a novel battery to study what happens in the electrode materials while it is actually operating (Bianchini M et al 2013; 2014). The aim was to obtain a high-quality, real-time movie of the entire process. To make the movie we created many neutron diffraction images by scattering neutrons through the electrode material as the battery charged and discharged. We then used neutron diffraction patterns as frames for the movie; much like stop-motion animation or making a flip book, playing the images one after the other allows us to see what’s really happening to the electrode.
We first tested and optimised the function of a single battery cell, the methodology and the quality of the diffraction patterns using relatively well understood electrode materials such as lithium iron phosphate (LiFePO4). LiFePO4 is already available as a safe, cheap and long-lasting electrode. Once satisfied with the experiment, we then studied some newer electrode materials based on lithium manganese oxide (LiMn2O4) to see how their behaviour differed. LiMn2O4 is an interesting positive-electrode material with a high capacity and charge/discharge rate, although its capacity quickly fades upon cycling, thwarting commercial application. By tweaking the molecular formula of the material, we found that adding more lithium to the structure, at the expense of manganese, can overcome this challenge.
Basing our work on the molecular formula of LiMn2O4, we made three compounds with the formula Li1+xMn2-xO4, where x is the amount of extra lithium. The three compounds had x values of 0, 0.05 and 0.10. By watching how the materials changes during charging, we found that the extra lithium reduces the usable capacity of the battery but eliminates the problem of fading capacity. The battery doesn’t hold as much charge to start with but it lasts longer at that same level.
We found that this is because:
- The volume change induced by the loss of lithium ions is reduced as the amount of lithium in the electrode material is increased, and the physical mechanism by which this happens is modified.
- When the amount of lithium is increased, the amount of Mn3+ is reduced by an equivalent amount, and we know that this species hinders the long life of batteries.
As a result, the material with the highest amount of lithium is a much better battery material.
We have not only shown that neutron diffraction can help us to understand the behaviour of lithium ions and lithium-ion batteries, but also found a real example of how to improve these batteries. Given our promising results, the study of this type of material is expanding to different compositions such as those containing additional nickel (LiMn1.6Ni0.4O4), operating at higher voltages and thus having more energy. Maybe one day our work will help your electric car run faster and last longer.
The transparent battery
The special battery cell that we used for this experiment has larger than normal electrodes so we could obtain the highest quality neutron diffraction patterns for analysis. The most important feature in this special battery is the use of a titanium–zirconium alloy, which is known for being neutron-transparent – it doesn’t scatter neutrons and instead they pass straight through. In this way, only the signal from the electrode of interest was collected, avoiding other unwanted contributions from the cell. We also used a deuterated version of the electrolyte, with hydrogen atoms replaced by the heavier deuterium, to further reduce scattering and improve the signal obtained.
Despite the larger than average size of the battery, the samples we had to study were still small (≈200 mg) in terms of what is normally required for neutron diffraction experiments (≈1 g). But thanks to the high power of the ILL neutron source and the state-of-the-art diffractometers available, we could get the images we were hoping for.
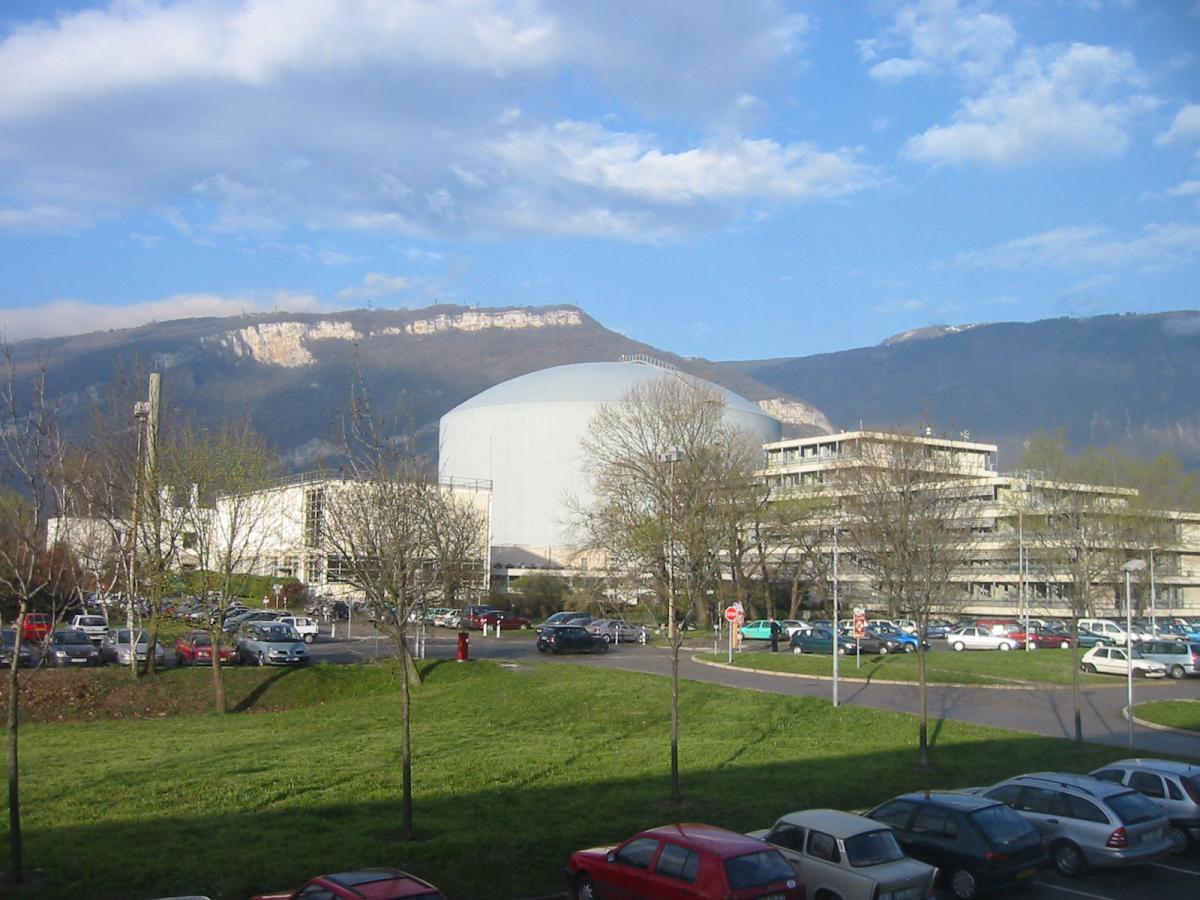
Image by Wurzeller [Public domain]; image source: Wikimedia Commons
More about ILL
The Institut Laue-Langevin (ILL)w1 is an international research centre based in Grenoble, France. It has led the world in neutron-scattering science and technology for more than 40 years, since experiments began in 1972. ILL operates one of the most intense neutron sources in the world, feeding beams of neutrons to a suite of 40 high-performance instruments that are constantly upgraded. Each year 1200 researchers from more than 40 countries visit ILL to conduct research into condensed matter physics, chemistry, biology, nuclear physics and materials science.
ILL is a member of EIROforumw2, the publisher of Science in School.
References
- Bianchini M et al (2013) A New Null Matrix Electrochemical Cell for Rietveld Refinements of In-Situ or Operando Neutron Powder Diffraction Data. Journal of the Electrochemical Society 160: A2176–A2183. doi: 10.1149/2.076311jes
- Bianchini M et al (2014) Li-Rich Li1+xMn2–xO4 Spinel Electrode Materials: An Operando Neutron Diffraction Study during Li+ Extraction/Insertion. Journal of Physical Chemistry C 118: 25947–25955. doi: 10.1021/jp509027g
Web References
- w1 –Learn more about ILL.
- See the list of all ILL-related articles in Science in School.
- w2 – EIRO forum is a collaboration between eight of Europe’s largest inter- governmental scientific research organisations, which combine their resources, facilities and expertise to support European science in reaching its full potential. As part of its education and outreach activities, EIROforum publishes Science in School.
Resources
- Scientists at the European Synchrotron Radiation Facility (ESRF) in Grenoble, France, also work on lithium-ion batteries and there is an interesting article on their work on pages 16 and 17 of the July 2015 issue of ESRF news.
Institutions
Review
Lithium-ion batteries are one of the most important electrochemical applications present in the daily lives of students. However, their low capacity and durability are factors that have delayed implementation, for example in electric vehicles.
Scientists at the Institut Laue-Langevin (ILL) explain in this article some of their work to improve characteristics of lithium-ion batteries. Teachers can use the article not only to give examples of cutting-edge research, but also to explain in class the basic concepts of electrochemistry which underlie the operation of batteries in general.
Several comprehension questions could be devised, regarding for instance the direction of the electrical current, or the electron flow; the function of the electrolyte; or even the stoichiometry of ionic compounds. The article can be used to discuss the difficulties that arise during the development of a technological product using the example of the durability of batteries vs capacity. It allows a connection between materials science and electrochemistry. Environmental issues may also arise with respect to the disposal of batteries.
Duarte Nuno Januário, Portugal