The secret life of volcanoes: using muon radiography Understand article
How do we find out what’s going on inside a volcano? Using cosmic rays!
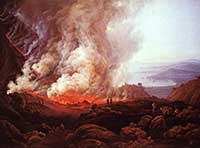
Johan Christian Dahl
(1788–1857)
Public domain image; image
source: Wikimedia Commons
Volcanoes are fascinating mountains, combining beauty with hidden danger. In the vicinity of an active volcano, such as Mount Vesuvius in Naples, Italy, people feel its presence like that of a gigantic living being that could attack at any time.This affects their attitude towards life: life is beautiful but unpredictable. How long will it be before Vesuvius erupts again? And what is happening deep inside the volcano?
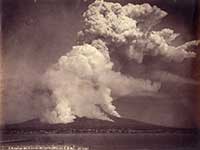
town of Naples, Vesuvius
erupted dramatically in 1872.
Photograph by Giorgio
Sommer (1834-1914).
Public domain image; image
source: Wikimedia Commons
We now have tools that go beyond imaginative speculation to find out exactly what is happening inside volcanoes, but unfortunately they are still very limited. Current methods are indirect. For example, one method uses small explosions to propagate small tremors around a volcano, which yields information from the way that these artificial seismic waves are reflected (like echoes) by rocks of different density. Using complex mathematics, this data can provide details of the internal structure of the volcano.
A new imaging technology
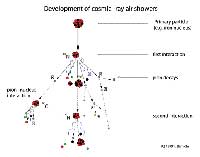
produced when a cosmic ray,
a primary particle accelerated
by mysterious mechanisms in
the distant Cosmos, reaches
us and interacts with an
atomic nucleus in Earth’s
atmosphere. Muons are
indicated by the symbol μ;
other particles shown are
photons (γ), pions (π),
neutrinos (ν) and energy (e).
Click on image to enlarge.
Image courtesy of the Max
Planck Institute for Astronomy
The aim of our project, which is a collaboration between scientists in Italy, France, the USA and Japan, is to further develop a new way to ‘see’ inside volcanoes directly. We want to produce shadow pictures, similar to the way that X-rays allow us to see inside the human body. But instead of X-rays, we are using muons (penetrating particles with a mass about 200 times that of electrons) – hence the project name of Mu-Ray. The technique is known as muon radiography.
Muons are produced, along with other particles, when cosmic rays (high-energy particles originating in outer space) interact with atomic nuclei in Earth’s atmosphere to produce ‘showers’ of secondary particles. The muons inherit the high energy of the parent cosmic rays, which enables them to penetrate and pass through the rock of the volcano and to be detected on the other side of the mountain. Because denser materials absorb more muons (just as dense materials such as bone absorb more X-rays), this provides a basis for producing shadow images of the volcano’s interior.
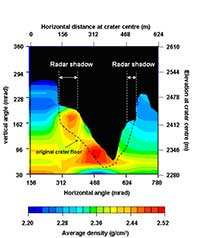
radiography of Japan’s
Asama volcano. The different
rock densities are shown on
a colour scale, and the
internal shape of the volcano
can be clearly seen. Click on
image to enlarge
Image courtesy of H T M
Tanaka
Muon radiography was first used in 1971 – not for volcanoes, but for investigating the interior of the pyramid of Chefren at Giza, Egypt. The Nobel-prize winning physicist Louis Alvarez placed a muon detector inside the pyramid to pick up changes in muon flux (rate of muon flow) that could indicate the presence of a hidden burial chamber. However, none was found.
In 2007, Hiroyuki Tanaka and collaborators from the University of Tokyo were the first to apply this technique to volcanoes. They carried out radiography of the top part of the Asama volcano in Honshu, Japan, which revealed a region with rock of low density under the bottom of the crater. The presence of low-density regions can be used in computer simulations that predict how possible eruptions could develop, indicating the most dangerous areas around the volcano. Their observations showed that muon radiography could indeed produce useful images of the internal structure of volcanoes.
The really important advantages of muon radiography of volcanoes are two-fold. First, whereas current indirect methods can provide information to a spatial resolution of some 100 m, muon radiography can be up to ten times more specific, mapping internal structures to a resolution of some 10 m. Second, muon radiography offers the possibility of continuous monitoring, thus potentially revealing the evolution of structures over time. The time resolution depends on the thickness of the rock traversed by the muons: the thicker it is, the fainter the muon flux and the longer it takes to accumulate enough muons for a picture. The time needed can thus be weeks, months or years.
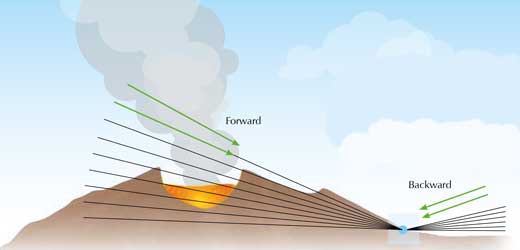
are absorbed by the rock through which they are passing. The denser the rock, the
more muons are absorbed. Backward flux is used for normalisation.
Image courtesy of Nicola Graf
Muon radiography is now being used for volcanoes around the world: in the Lesser Antilles, at the Puy de Dôme in Central France, and in our very challenging work on Vesuvius with the Mu-Ray project. The images are produced using detectors called muon telescopes, which use technology developed in particle physics and play the role of the X-ray film in conventional radiography. The telescopes detect near-horizontal muons emerging from the volcano’s edifice, having passed right through it. By reconstructing the path of each single muon through the volcano, the apparatus reveals the amount of muon absorption in each direction. Denser rocks absorb more muons, so a map of muon fluxes gives a negative image of the rock densities inside the volcano. Such images cannot help to predict when an eruption might occur, but – combined with other observations – can help to foresee how one could happen.
Imaging Vesuvius
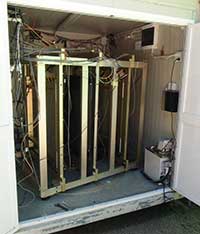
prototype at Vesuvius.
Image courtesy of Paolo Strolin
So, what about Vesuvius? This volcano is a special challenge, not only because it represents the highest volcanic risk in Europe, but also because of the mountain’s unusual structure. Vesuvius is in fact situated within the remnants of a much larger volcano, Mount Somma. Moreover, inside the summit of Vesuvius is a crater that is 500 m wide and 300 m deep: this means that, to look below the bottom of the crater, muons have to penetrate deep into the mountain, through almost two kilometres of rock, to reach the detector on the opposite side of the volcano. Only muons of very high energy travelling in a near-horizontal direction are able to pass through all this rock, so their flux at the detector is very low, making imaging extremely difficult. This explains why the project – and the development of muon radiography – is extremely challenging.
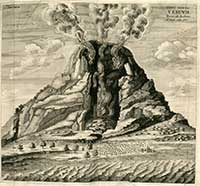
according to Athanasius
Kircher (1602-1680), from
his work Mundus
Subterraneous
Public domain image; image
source: Wikimedia Commons
To look inside Vesuvius, therefore, we need to develop a new type of muon telescope. To detect enough particles of such low flux to produce an image, this apparatus must cover a much larger area than previous muon telescopes. Substantial improvements are also needed to distinguish the experimentally important particles from background muons – which we plan to do by measuring each muon’s time of flight through the telescope to confirm that it really has the right direction to have passed through the volcano.
A prototype telescope with a detector area of just 1 m2, compared to 10 m2 or more to be covered by the final telescope array, has been recording data at Vesuvius since Spring 2013. The data is currently being analysed. The detectors consist of plastic scintillator strips – a technology borrowed from particle physics. These strips can be used to cover large areas and provide long exposure times, and they are robust enough to withstand volcanic conditions. Of practical importance too are the telescope’s low energy consumption, enabling it to be powered by a solar panel, and its portability, so that it can be used in different locations. Depending on funding and the experience gained with the prototype, we hope as the next step to construct two telescope arrays, each with total areas of 4 m2, to record data for one year or more.
New frontiers
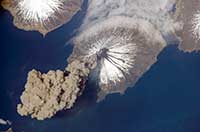
Volcano, Aleutian Islands,
taken from the International
Space Station on 23 May
2006. The volcano emitted a
plume of ash but did not
erupt.
Image courtesy of NASA
Meanwhile, particle physicists and volcanologists continue to work together in muon radiography. As well as providing a powerful tool for the study of geological structures, this expanding field also has potential industrial applications, such as seeing inside nuclear reactors or determining the remaining thickness of the wall of an iron furnace, which can then be replaced at the right time.
Alongside these possibilities, there is another developing technology that promises imaging on an even larger scale: neutrino radiography. With their extraordinary penetration power, neutrinos produced by cosmic rays and passing through Earth itself could at some future date provide information about the density of the core of our own planet.
Acknowledgments
The Mu-Ray project is funded by the Istituto Nazionale di Fisica Nucleare (Italian national institute for nuclear physics) and the Istituto Nazionale di Geofisica e Vulcanologia (Italian national institute for geophysics and volcanology), with contributions by the Italian Ministry of Education and Research (MIUR-PRIN), Fermilab (USA) and IN2P3-Orsay (France) and support from the Provincia di Napoli (province of Naples) and the Istituto Fondazione Banco di Napoli (foundation of the bank of Naples).
The author would like to gratefully acknowledge the contributions to this new field of research by HKM Tanaka and A Taketa (ERI-Tokyo); K Niwa and T Nakano (Nagoya); D Gibert and J Marteau (DIAPHANE collaboration); C Carloganu (TOMUVOL collaboration); F Ambrosino, G Castellini, R D’Alessandro, G Iacobucci, M Martini, M Orazi and G Saracino (Mu-Ray collaboration).
Web References
- w1 – Learn more about the Scienza e Scuola (science and school) project.
Resources
- To learn more about the Mu-Ray project
- Details can also be found in: Poppi F (2011) Muons reveal the interior of volcanoes. CERN Bulletin 50:2
- For more technical details about the Mu-Ray project, see:
- Anastasio A et al. (2013) The Mu-Ray experiment: An application of SiPM technology to the understanding of volcanic phenomena. Nuclear Instruments & Methods in Physics Research A: Accelerators, Spectrometers, Detectors and Associated Equipment 718: 134-137. doi: 10.1016/j.nima.2012.08.065
- Ambrosi G et al. (2011) The Mu-Ray project: Volcano radiography with cosmic-ray muons. Nuclear Instruments & Methods in Physics Research A 628(1): 120-123. doi: 10.1016/j.nima.2010.06.299
- Beauducel et al. (2008) Muon radiography of volcanoes and the challenge at Mt. Vesuvius. The article can be downloaded from the Mu-Ray project website (click on ‘Read the complete proposal’).
- The website of the European Space Agency (ESA) offers multimedia background information about cosmic rays.
- ESA’s Eduspace website offers school-level information about volcanoes, including how they are monitored.
- To mimic in the classroom how volcanoes are traditionally investigated, by monitoring the characteristic way seismic waves travel through rocks of different density, see:
- Bazanos P (2012) Building a seismograph from scrap. Science in School 23: 25-32.
- With its most famous eruption, in 79 AD, Mount Vesuvius destroyed the Roman town of Pompeii. To learn how modern scientific analyses are casting light on the ancient town, see:
- Capellas M (2007) Recovering Pompeii. Science in School 6: 14-19.