How plants beat jet lag Understand article
New research is shedding light on the internal ‘clocks’ that help plants respond to changing day-night cycles.
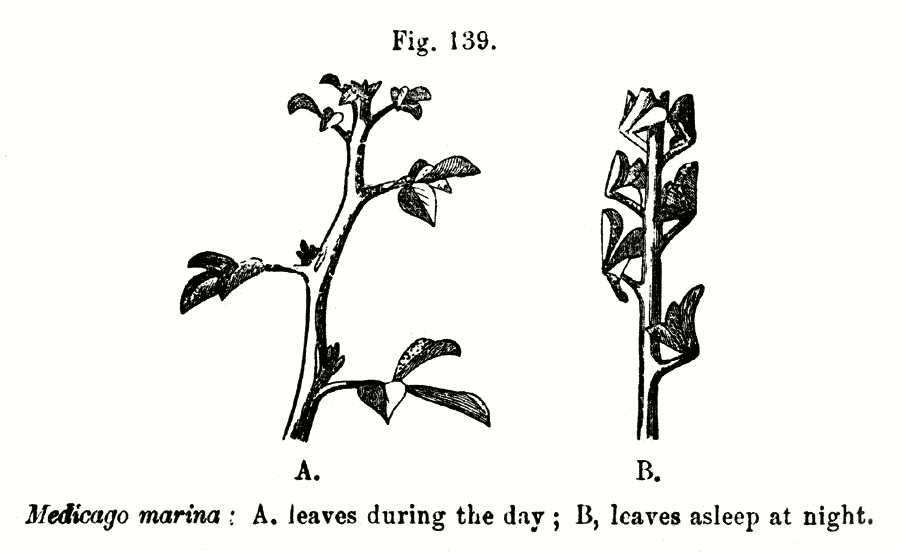
Medicago leaves from Charles Darwin’s
book The Power of Movement in Plants
Charles Darwin (1898)/Wikimedia Commons,
public domain
Have you ever experienced the disorientating feeling of jet lag after a long-haul flight? The reason it feels so awful is a result of the mismatch between the environment and our internal body clock: sunlight tells us it’s the middle of the afternoon, but our internal clock tells us it’s time to sleep. This clock – also known as the body’s circadian clock, or circadian oscillator – controls our body’s biological rhythm. It is an evolutionary adaptation to living on a rotating planet where light and temperature levels fluctuate over a 24-hour period, and it affects our sleep cycle, our metabolism and other aspects of our physiology. The word ‘circadian’ comes from the Latin ‘circa’, meaning around, and ‘dies’, meaning day.
Circadian rhythms were first observed in plants, with the earliest account made in the 4th century BCE when an admiral of Alexander the Great’s fleet described the daily rhythms of tamarind leaves. Charles Darwin described leaf ‘sleep movements’ in his 1880 book The Power of Movement in Plants, after observing that the leaves of Medicago plants fold up at night. So if plants have body clocks, does this mean that a plant could suffer from jet lag?
Testing for circadian rhythms
Since our circadian rhythms are controlled by an internal clock, the rhythms persist even when the external conditions (such as light) are constant. This makes them more complex than diurnal rhythms, which are simple responses to external conditions that no longer occur when the conditions are constant. Most daily rhythms are in fact circadian rhythms, but there is a simple test to check whether a biological process is a genuine circadian rhythm or not. Scientists first expose an organism (either plant or animal) to 24-hour cycles of stimuli (e.g. 12 hours of light, followed by 12 hours of darkness). Then, the organism is put into continuous conditions (e.g. constant light) and the process is measured for several days. If the rhythm (such as the sleep-wake cycle or body-temperature cycle) persists even though the conditions are constant, it is controlled by an internal body clock and is not just a response to fluctuating environmental conditions (figure 1). This test has been performed in many different organisms, so we now know that mammals, insects, plants and even some bacteria have genuine circadian clocks.
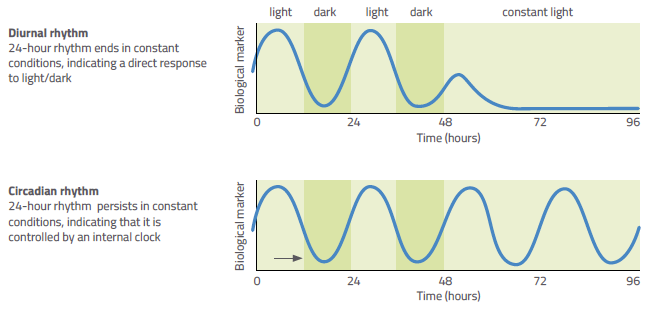
Katharine Hubbard
Plants keep track of time
We now know that the circadian clock controls almost all aspects of plant biology, including growth, flowering, photosynthesis, and the opening and closing of leaf stomata. In most plants, the stomata open just before dawn so the plants can start photosynthesising as soon as light is available. Plants also use their internal clocks to ‘measure’ day length, which determines a plant’s flowering time. For example, some short day plants (e.g. rice, chrysanthemums) only flower when the daylight is shorter than a critical length. Chrysanthemums, for instance, will start to flower if the day is shorter than their critical day length of 15 hours, so tend to flower in the spring or autumn. Long day plants (e.g. lettuce, spinach) have the opposite response, so will only flower when the day exceeds a critical length during the summer. In contrast, day neutral plants are not sensitive to the number of hours of light. Short-day and long-day plants with a faulty circadian clock flower earlier or later than they would do normally, as they are unable to determine the day length. Non-functioning clocks can even affect plants’ defence mechanisms, preventing them from synthesising defence compounds at the right time of day, making them more prone to attack by insects.
Circadian clocks thus have important implications for agricultural productivity. Experiments using the model plant Arabidopsis demonstrate that, in controlled laboratory conditions, plants with a non-functional circadian clock grow less well than plants with a functioning clock. This is because plants with a non-functional clock make less chlorophyll and have lower photosynthetic rates (Dodd et al., 2005).
As the clock controls flowering time, it also dictates harvest time for crop species. For example, the barley that is grown in southern Europe is sensitive to day length, so it flowers early in the spring and is harvested in early summer before it gets too hot. In northern Europe, however, the climate is much cooler; the barley grown there has a natural mutation that affects the way that the clock regulates genes controlling flowering time, making the plant less sensitive to day length. This mutation allows the plant to take advantage of the longer summer days so it can be harvested in the autumn. As a result, there is increasing interest in the circadian clock from biotechnology companies hoping to increase crop yields by manipulating the circadian clock.
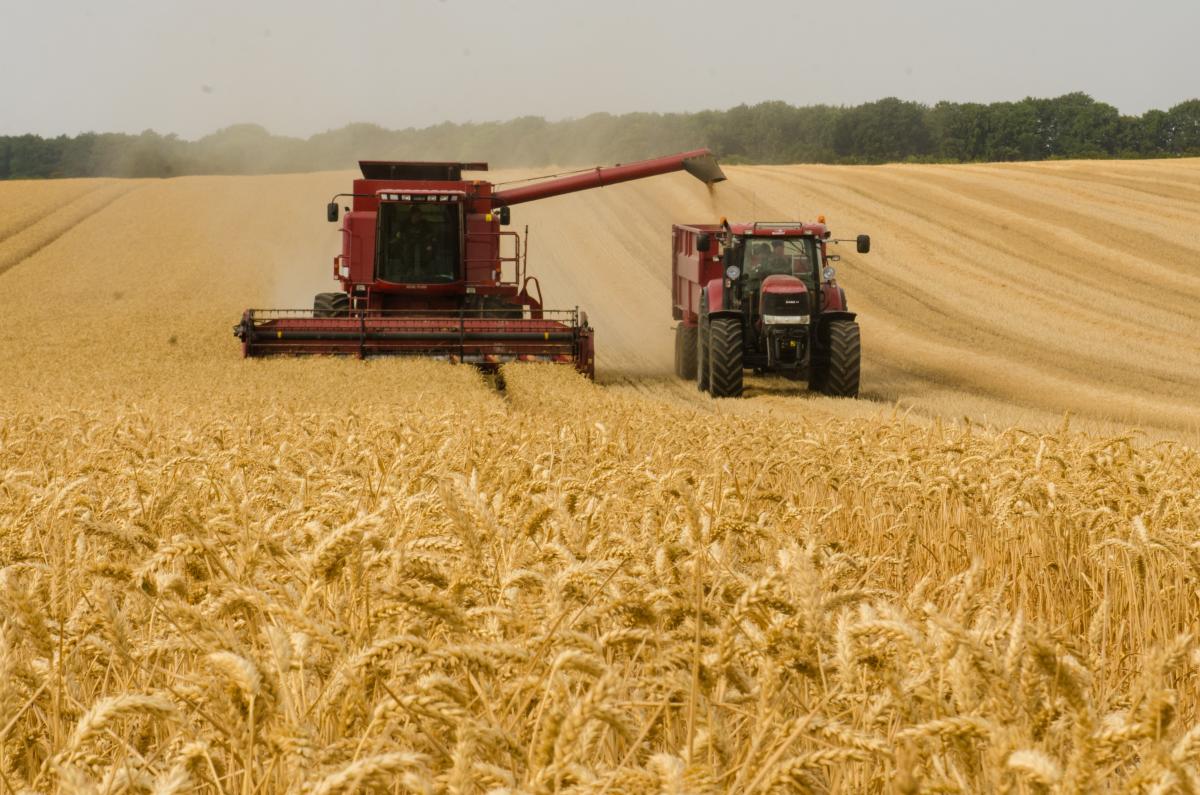
stanvpetersen/pixabay.com
Genetics of circadian rhythms
So how does circadian regulation work? The answer lies in the plant’s genetics. The clock consists of many genes that control each other in a type of self-regulating system known as a negative feedback loop. Figure 2 illustrates how this genetic regulation works using a circadian clock with two genes. Gene A is switched on (e.g. through a light-dependent process) and produces protein A. Protein A then activates gene B, resulting in the production of protein B. Protein B represses the expression of gene A, which means that levels of protein B decrease, allowing gene A to be expressed again. This results in a cycle where protein A is produced at the opposite time to protein B. If each of these regulatory steps takes 12 hours, the result is a 24-hour rhythm that can sustain itself in constant conditions (e.g. 24 hours of continuous light).
In reality, the mechanism behind circadian rhythms is much more complicated than this: the plant circadian clock comprises a network of over 20 genes and their related proteins (McClung, 2019). The clock is so complex that biologists are struggling to understand all of the connections and make predictions about what will happen if the network is disturbed. So to get to grips with the problem, biologists have teamed up with mathematicians to create computational models of the circadian clock, which are now being used to help with the design of experiments. For example, models have been built that integrate information about light levels, CO2 availability and temperature with models of circadian gene expression, which are then used to predict the growth rate of the plant under different day lengths.
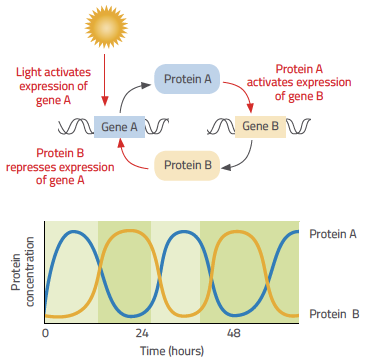
Katharine Hubbard
Avoiding jet lag
One of the major differences between plant and human circadian clocks is the degree of central control. Our circadian clock is coordinated by an area of the brain called the suprachiasmatic nucleus (SCN), which is located in the hypothalamus. During the evening, the SCN tells the pineal gland in the brain to produce melatonin, which then travels through the body preparing other organs, such as the stomach and liver, for sleep. Since our circadian clock largely relies on hormones circulating through the bloodstream, any circadian responses are relatively slow. When someone is jet lagged, different parts of their body end up being set to different time zones. It can take a few days after a flight for all areas of the body to adopt the same time again.
This is quite different to a plant’s circadian clock, which is thought to function at the level of individual cells. Each cell in the plant has its own light receptors and circadian clock, so unlike in humans, individual cells can respond to light. If you cover up parts of a plant with tin foil, you can train one part of the leaf to think it is daytime and another part to think it is night-time. This difference in clock architecture means that plants can more easily correct their circadian rhythms, so if a plant were to be flown halfway around the world, it would be much better at coping with the time difference than we would and, overall, jet lag wouldn’t be a major issue. Unlike in humans, plants could combat jet lag in a matter of hours rather than days.
Whether plants can adjust to new time zones might be an interesting biological curiosity, but understanding the underlying biology of plant circadian rhythms has taught us a lot about how genetic networks are regulated – which ultimately could help improve agricultural yields in the future.
References
- Dodd AN et al. (2005) Plant circadian clocks increase photosynthesis, growth, survival, and competitive advantage. Science 309: 630–633. doi: 10.1126/science.1115581
- McClung CR (2019) The plant circadian oscillator. Biology 8: 14. doi: 10.3390/biology8010014
Resources
- For time-lapse videos showing plant circadian rhythms in various species, visit the Plants in Motion website.
- Read a short textbook introducing circadian rhythms in humans and their impact on our wellbeing. See:
- Foster R, Kreitzman L (2017) Circadian Rhythms: A Very Short Introduction. Oxford, UK: Oxford University Press. ISBN: 9780198717683
- The American Society for Plant Biology has published teaching resources on circadian rhythms. Although intended for undergraduate students, the resources could complement school-level classes or form inspiration for projects. See:
- Hubbard KE, Dodd AN (2016) Rhythms of life: the plant circadian clock. The Plant Cell 28. doi: 10.1105/tpc.116.tt0416
- Read a special issue of the journal Biology that celebrates the 2017 Nobel Prize in Physiology or Medicine, which was awarded to three biologists for their discoveries of the molecular mechanisms that control circadian rhythms.
Review
Have you ever wondered why some plant species flower in the winter and others flower in the summer? Or why we sleep at night and stay awake during the day? The answers to these questions lie in the circadian rhythm, a process that occurs in both animals and plants and is also responsible for jet lag. This article explores the biology of the circadian rhythm in a clear and precise manner, and considers the role of genes and hormones in regulating our daily rhythms. In addition to exploring the comprehension questions below, the article could be used to more broadly discuss the effects of environmental conditions on plant productivity, and the impacts of climate change and environmental disturbances on circadian rhythms.
Possible comprehension questions could include:
- What is the difference between circadian and diurnal rhythms?
- Why do different plants flower in different seasons?
- How can genetic mutations influence agricultural productivity?
- Why can plants correct their circadian rhythm more quickly than humans can?
Monica Menesini, science teacher, Italy