Heroes and villains: the science of superheroes Teach article
Challenge your students to work out which exploits of comic-book heroes like Superman might actually be possible – given a miracle or two.
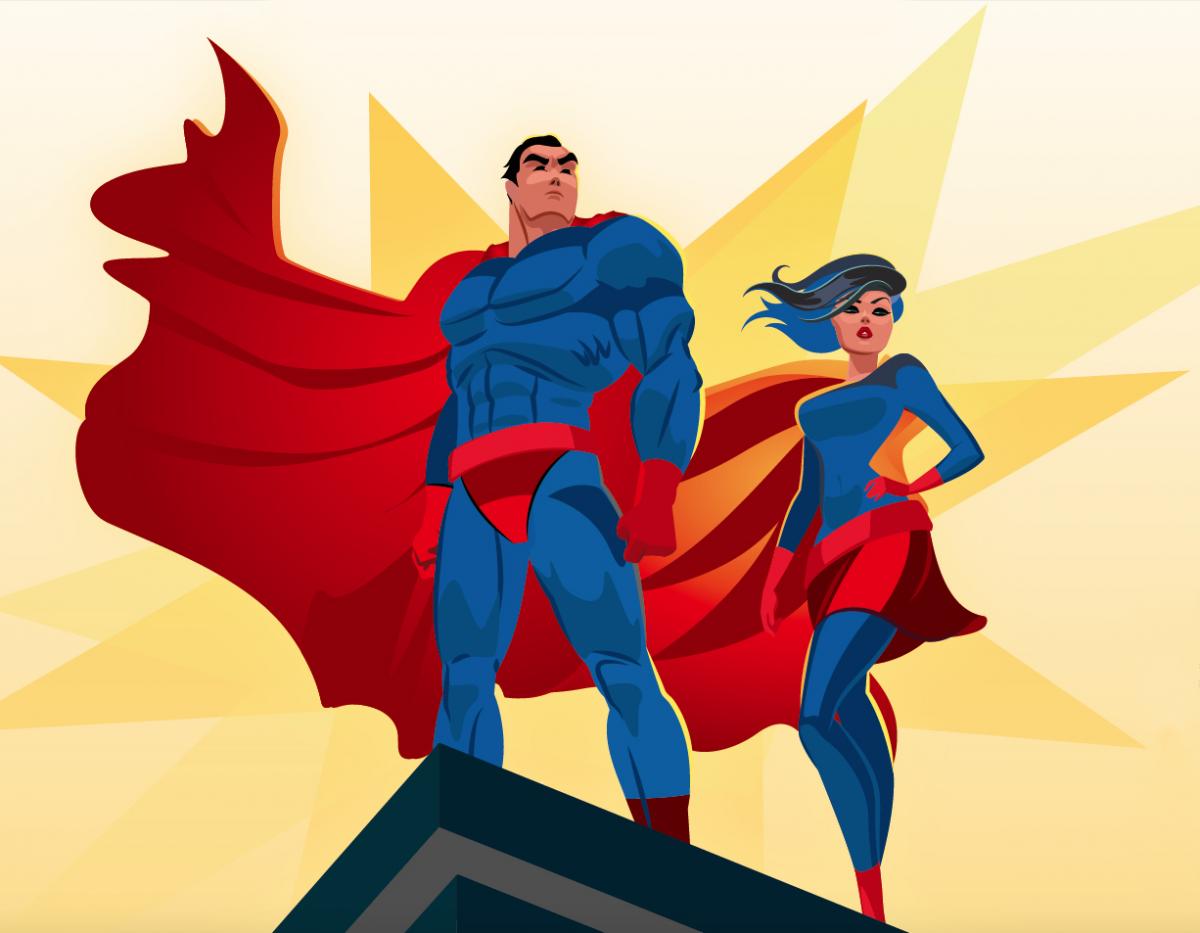
Maksutov / Shutterstock.com
As a trained scientist, you probably enjoy applying your scientific knowledge to everyday topics, because of the insight this provides into how the world works – and the subtlety involved in deciding which principles to apply.
As a physics teacher, I wanted to allow my students a similar freedom to break out of the school curriculum for a while and consider the science in situations that are intrinsically interesting to them – if not exactly everyday. Prompted by the book The Physics of Superheroes (Kakalios, 2009), I decided to try incorporating comic-book superheroes into my physics lessons to make the learning experience more engaging for students. The experiment was a success, and while my classes expressed a real enthusiasm for the topic, they also got down to work on some quite challenging science.
In this article, I describe some of the materials I used with my classes, including questions and worked answers, plus some background information on the superheroes themselves for those less acquainted with these colourful characters. These materials are suitable for students aged 16–19, or as a challenge for some able younger students. Discussions and calculations could occupy a class for an hour or longer.
What’s science got to do with it?
Superheroes can perhaps best be used in lessons as problem-solving examples, where real science is applied to the heroes’ proclaimed abilities to test how coherent these are, and what consequences follow logically from their supposed superpowers. As with any fiction, appreciating comic-book superheroes demands some suspension of disbelief. But for superheroes that are based on science fiction (as opposed to pure fantasy), if we grant them what James Kakalios calls one or more ‘miracle exceptions’, their powers may become plausible. For example, if we allow that Superman can leap tall buildings in a single bound, what does this tell us about the force of gravity on his home planet, Krypton? We will look at this question later.
Another possibility is to look at a specific superpower and use this as a context for describing or explaining science. Below I discuss some possibilities based on Marvel Comics’ X-Men characters, and also that most enduring superpower: invisibility.
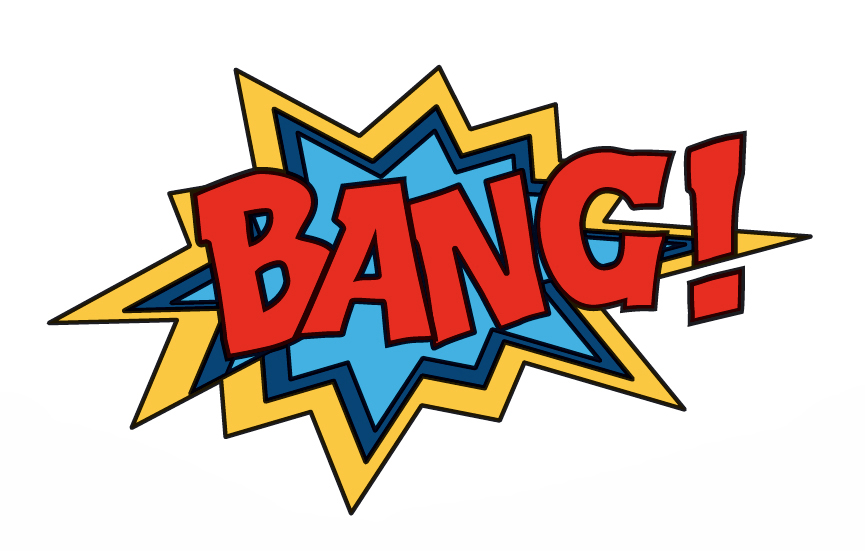
X-Men and their superpowers
The X-Men team of superheroes was created in 1963 by Marvel Comics editor Stan Lee and artist/co-writer Jack Kirby. The X-Men are a mutant subspecies of human who are each born with a different superpower. Let’s look at some of the main characters.
- Charles Xavier, the leader of the X-Men, is also known as Professor X. Confined to a wheelchair, he has the superpower of telepathy. A class discussion on whether this is plausible could focus on the nature of nerve impulses, and the fact that brain activity involves ions moving within the neural network of our brains. This links to electromagnetism, as the moving ions constitute an electric current that generates a magnetic field, causing low-frequency electromagnetic waves to be radiated from the brains. In principle, it might be possible to ‘read’ someone’s mind – although how detailed this could ever be is an open question.
- Magneto, the arch-villain of the X-Men, is a mutant who can generate and control magnetic fields. This superpower provides an opportunity to introduce the different forms of magnetism: ferro-, para- and diamagnetism. The class could also look at how diamagnetism can produce levitation, and whether it would be possible to levitate a person (yes, if the magnet is strong enough: at least 40 tesla, which is some 40 times stronger than a junkyard magnet for lifting cars).
- Wolverine is a mutant with a powerful regenerative ability, known as the healing factor, and six retractable bone claws in his hands tipped with the indestructible material adamantium. This fictional metal alloy could prompt students to think about what an ‘indestructible’ material would be like. Materials have three basic mechanical properties: strong materials bear heavy loads; hard materials resist changing shape or being scratched; and tough materials resist fracturing, often by changing shape to absorb energy. For example, although spider silk is tough, it is not hard. While the covalent bonds within diamond make it extremely hard, it is not as tough. Students could be asked to research which real materials (natural or synthetic) excel in each of these properties and why, as well as what new, stronger materials are being developed.
The invisibility cloak
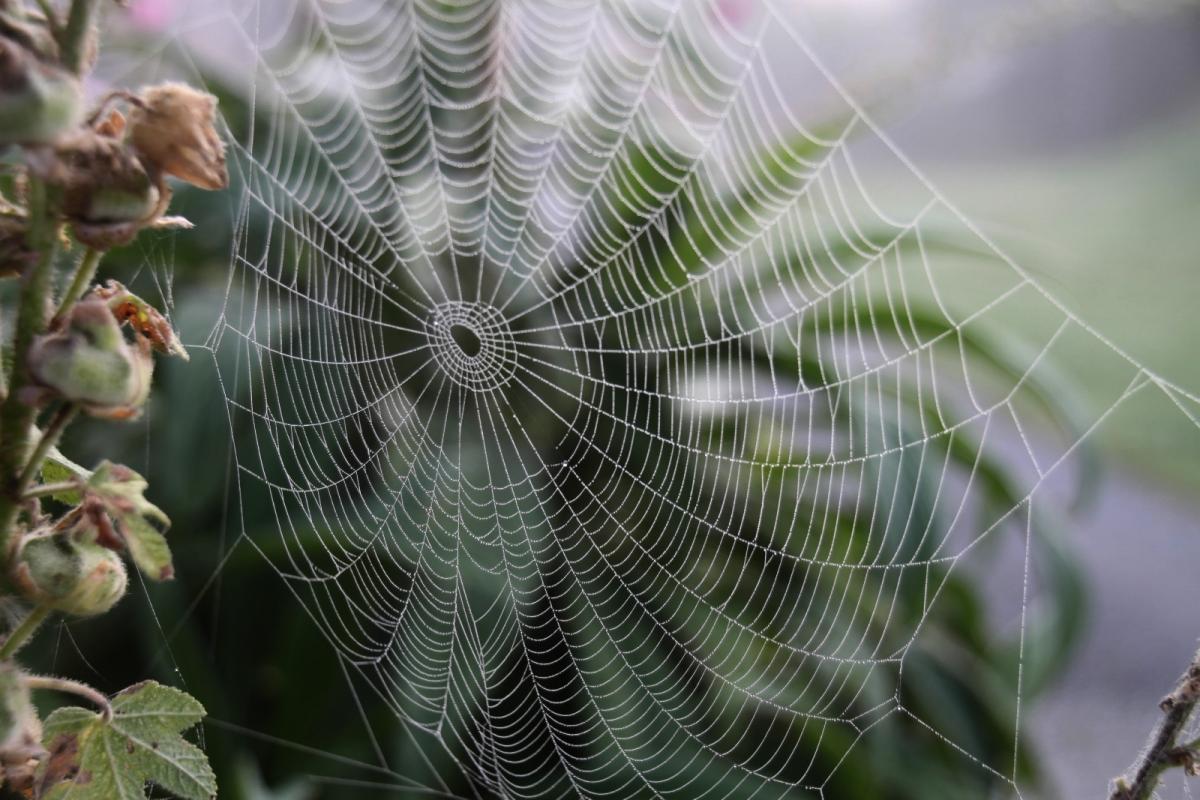
Image courtesy of Jeanne Menjoulet; image source: Flickr
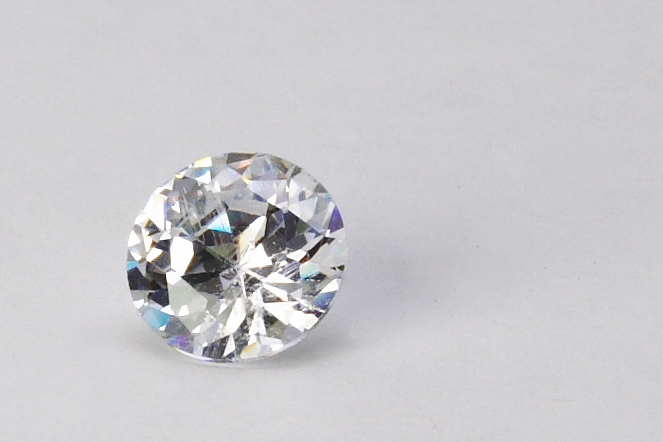
The idea of using a cloaking device to achieve invisibility was first suggested in a 1966 episode of Star Trek. Ask students to discuss: is this something that could actually be achieved? If so, how? What would need to happen for a cloak to make an object invisible? Perhaps ask students to draw ray diagrams that show how this could be achieved.
In principle, if light could be bent around an object, rather than reflected or refracted by it, the object could appear invisible. Metamaterials (real materials designed with properties that natural materials do not have) are now being developed to do just this, by allowing electromagnetic radiation to pass around a ‘cloaked’ object, like a river flowing around a rock. An observer looking at the cloaked object would then see light from behind it, making the object appear invisible. Although this approach shows promise, only tiny objects have been successfully cloaked so far.
Superman: an exercise in problem solving
Superheroes are a fruitful source of questions on the motion of projectiles and other physics topics. Superman made his debut in the first issue of Action Comics in 1938. Readers were informed that he could leap one-eighth of a mile (200 m) high, which can be used as a starting point for finding out other physical facts, if we add some plausible assumptions.
Below are some Superman questions for students to consider, along with their solutions.
1. If Superman jumps 200 m high, show that his launch speed is about 60 ms-1.
There are two possible approaches to this problem: via an equation of motion or via energy conservation. Ask students to solve the problem using both approaches, as this is a good technique for checking answers. It also illustrates what professional physicists do – if alternative approaches give consistent answers, this provides confidence in the result.
Equation of motion approach
Equations of motion used in school physics are often called ‘suvat’ equations, as they involve the following quantities:
s = displacement
u = initial velocity
v = final velocity
a = acceleration
t = time
Remind students that, if Superman jumps vertically upwards, when he reaches his maximum height he will come to a momentary stop, so his final velocity v = 0 ms-1. Assuming that upwards motion is positive, the values of the variables are:
s = 200 m
u = ?
v = 0 ms-1
a = -10 m-2 (acceleration due to gravity)
t = irrelevant
So the most suitable suvat equation is:
v2 = u2 + 2as
Using the values we have, we can see that:
0 = u2 + 2 (-10 x 200)
u2 = 4000
u = 63.2 ms-1
Energy conservation approach
We assume that, at the top of Superman’s jump, all kinetic energy has been transformed into gravitational potential energy. So:
1/2 mv2 = mgh
where m is the mass of Superman, v is his launch speed and h is the height he gains above the ground.
Simplifying and substituting values gives:
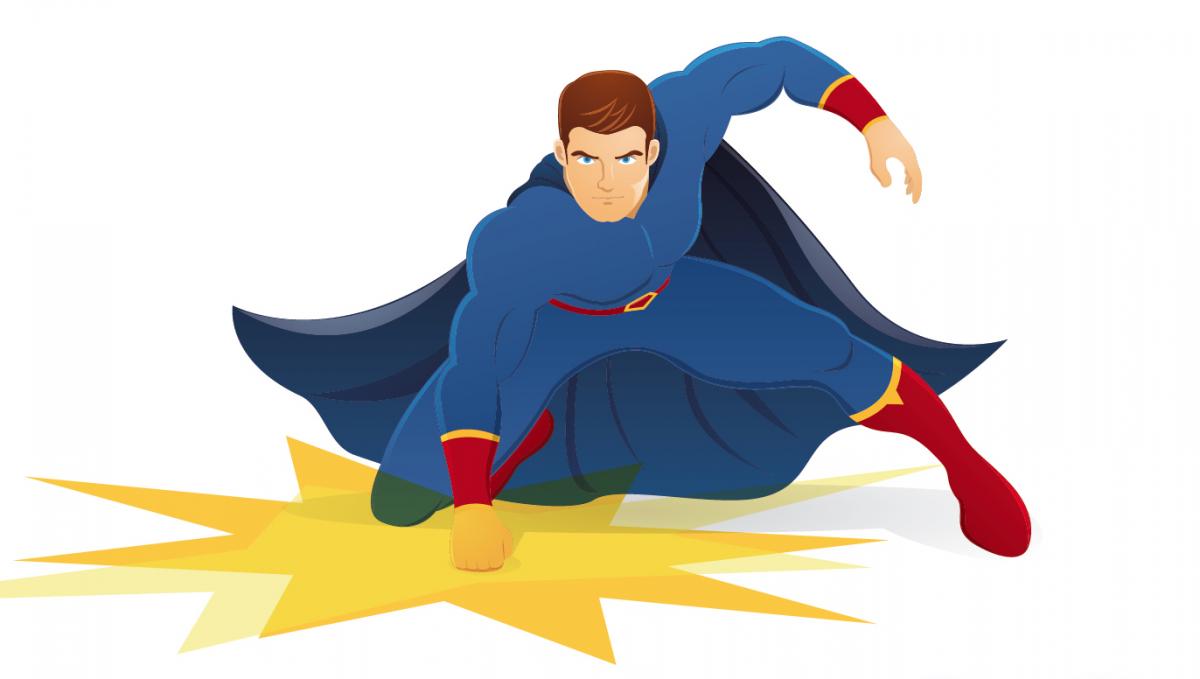
2. When he jumps, what force would Superman experience pushing off from the ground?
Force, F, is defined as the rate of change of momentum, p. Momentum is the product of mass and velocity, so the rate of change in momentum is Superman’s mass m multiplied by his launch velocity v, divided by the time taken, t.
Let’s assume Superman has a mass of 100 kg. For t, a standard value for ‘push-off time’ often used in textbooks is 0.25 s.
This gives us the following equation:
3. If Superman can jump 200 m on Earth, what is the likely gravitational strength of his home planet, Krypton?
When most people jump, they can apply a force approximately equal to their standing weight. On Earth, Superman’s standing weight, W, is his mass, m, multiplied by the gravitational field strength, g (which is approximately 10 Nkg-1). Superman has (we assume) a mass of 100 kg, so:
W = 100 kg x 10 Nkg-1 = 1000 N
From the question above, we know that when Superman jumps, he applies a force of 2.53 x 104 N, suggesting that this is his weight on Krypton. This in turn suggests that the gravitational field on Krypton is some 25 times stronger than it is here on Earth.
Superman was physiologically adapted for life on Krypton, with its much higher gravitational field strength. On Krypton, he would have seemed average; on Earth, he appears to have superpowers.
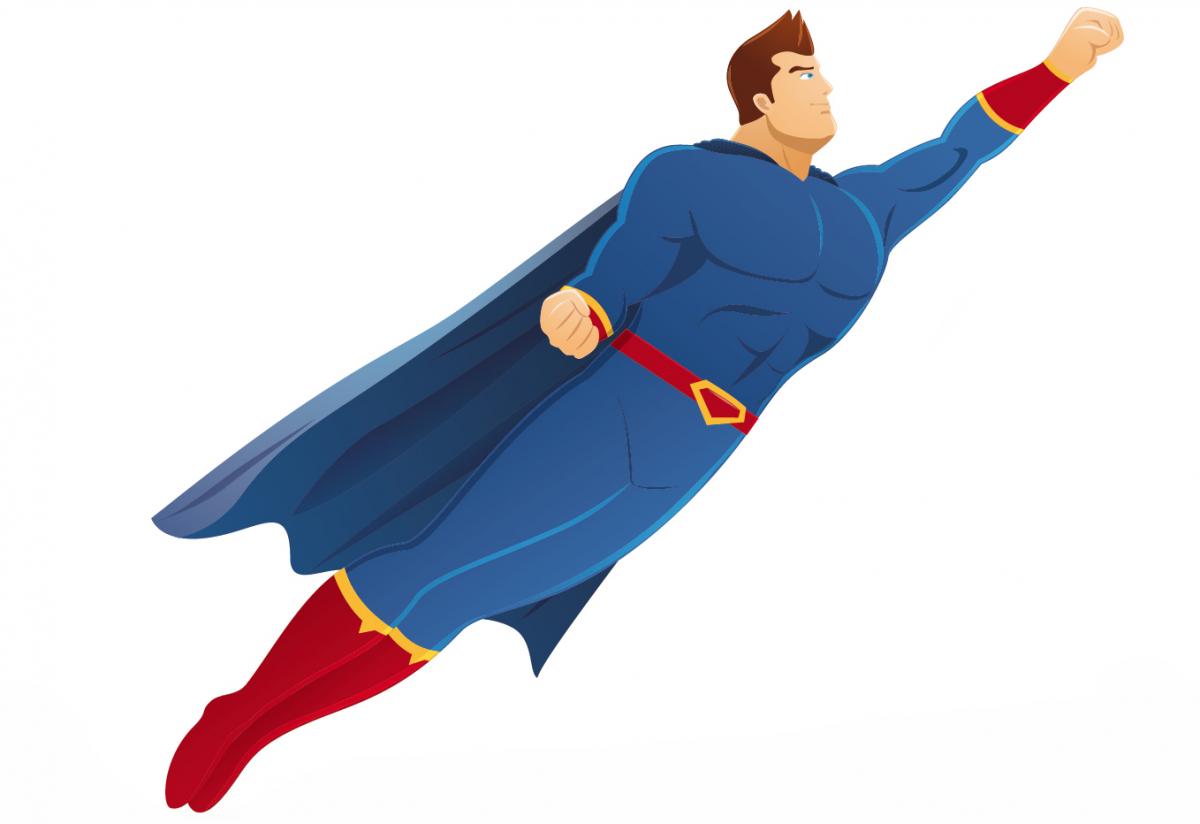
4. What can we deduce about Krypton from its gravitation?
This question could be used as a simple introduction to mathematical modelling for students aged 16+. The strength of a planet’s gravitational field is proportional to its radius, if the density is uniform. Students can find this relationship themselves, given the equations for gravitational field strength, g = Gm/r2 , and for the volume of a sphere, V = 4/3pr3.
This means that if Earth and Krypton have similar density and the strength of Krypton’s gravitational field is 25 times stronger than that of Earth, then the radius of Krypton is also 25 times that of Earth’s.
From this we can draw conclusions about Krypton’s mass. Again, assuming uniform density, the mass of a planet is proportional to the cube of its radius. Thus, a 25-fold increase in the Earth’s radius would increase its mass by a factor of 253 = 1.56 x 104.
Assuming a value of 5.97 x 1024 kg for the mass of Earth, this means that the mass of Krypton would be:
5.97 x 1024 kg x 253 = 9.33 x 1028 kg
This is a mass some 15 000 times that of Earth, and around 50 times that of Jupiter. In fact, if Krypton were a gas planet (rather than a rocky planet like Earth), this would be just a little below the mass required to allow the thermonuclear fusion of hydrogen – the process that produces the Sun’s energy. So if Superman’s creators had made him a little more mighty, his home ‘planet’ could in fact have been a star.
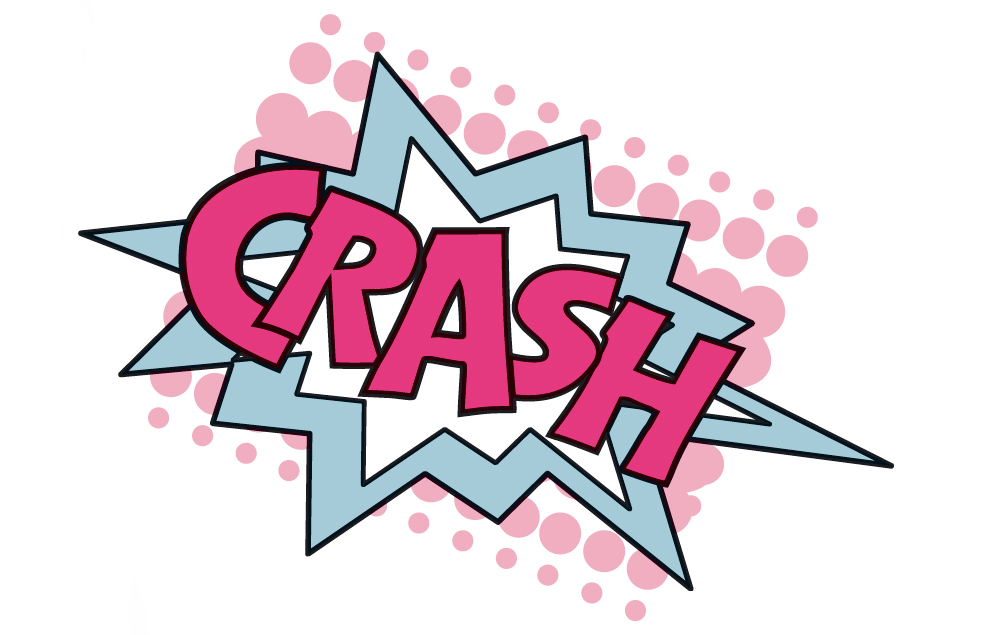
Ant-Man and other scenarios
It’s possible to come up with many similar questions and scenarios based on comic-book characters to exercise students’ scientific imagination. For example, the Marvel character and biophysicist Dr Henry ‘Hank’ Pym discovers a chemical substance (Pym particles) that makes him shrink to the size of an insect, becoming Ant-Man. When Ant-Man gets sucked up by a vacuum cleaner, would he be able to punch his way out of the bag?
This question is really an exercise in scaling. The force with which someone can punch is proportional to the cross-sectional area of muscles in their arms. But because the force of Ant-Man’s punch and the area of his fist are both reduced by the same factor, the pressure he could exert on the paper bag would – perhaps surprisingly – be the same as that for Dr Pym.
Of course, this requires the ‘miracle exception’ of Dr Pym’s volume shrinking while all other attributes (including mass) are kept constant – a scenario that leads us into a completely different area of science: atomic structure. Which shows that, with a little imagination, superheroes can take you anywhere. Enjoy the journey!
References
- Kakalios J (2009) The Physics of Superheroes 2nd edition. New York, NY, USA: Gotham Books. ISBN: 1592405088
Resources
- For a review of The Physics of Superheroes, see:
- Featonby D (2006) The Physics of Superheroes, By James Kakalios. Science in School 2: 81.
- For another book looking at the science associated with superhero characters, see:
- Gresh LH, Weinberg R (2002) The Science of Superheroes. Hoboken, NJ, USA: Wiley. ISBN: 0471024600
- Watch a video showing frogs being magnetically levitated.
- For information on metamaterials and invisibility cloaks, see:
- The website of the UK’s Institute of Physics.
- Invisibility shields one step closer with new metamaterials that bend light backwards, a press release from the University of Berkeley, USA.
- The student-run Journal of Special Physics Topics has papers on a huge range of fun, non-standard physics topics. Volume 14(1), for example, covers Superman, Santa and Cinderella.
- In entertaining videos, Kyle Hill describes and explains the science of superheroes.
Review
This teaching activity is ideal to show how the ‘superpowers’ of comic-book heroes and villains can be investigated (or proved to be impossible) using scientific facts. The article encourages teachers to look at the surreal adventures experienced by these characters from a new perspective. Some examples provide introductory exercises to use before starting a new topic, such as mechanics, magnetism, materials or light waves – or at the end of these same topics, so that the students can use their newly acquired scientific knowledge to theorise, predict and test whether such superpowers are remotely possible in real life.
Catherine Cutajar, St. Martin’s College, Malta