The challenging logistics of lunar exploration Understand article
The path to the Moon is paved with many challenges. What questions do the next generation of space explorers need to answer?
In the first of this two-part series, I explained why scientists wish to return to the Moon, what scientific questions remain and why it is important to find the answers (Tranfield, 2014). In this follow-up article, I describe some practical but non-trivial challenges to fulfilling a successful mission to the Moon, together with potential leads for solutions. Your students, the next generation of space explorers, can put their minds to work to generate ideas and solutions to these problems.
Where should we go on the Moon and why?
To the poles? The equator? The near side of the Moon? Its far side? The answer to this depends on which scientific questions are being studied. The Apollo missions all landed on the near side of the Moon, near the equatorw1. Scientists think new destinations should be explored to expand our understanding of the Moon. Destinations of interest include the poles, particularly the South Pole–Aitken Basin, which is the largest, oldest and deepest known crater on the lunar surfacew2, making it an interesting study location for lunar geologists.
Furthermore, NASA’s LCROSS mission in 2009 determined that there is ice at the poles in regions that never receive direct sunlightw3. Any experiments that study lunar ice will need to target the lunar poles. The far side of the Moon would also be an interesting new destination for exploration but it is technically more challenging because there is never a direct line of sight between the far side of the Moon and Earth, which would make mission control and communication more difficult.
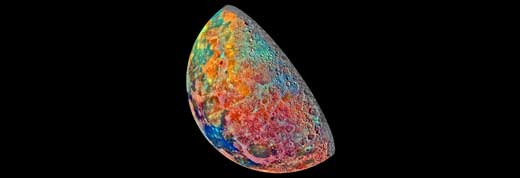
Bright pink: highland materials, such as those surrounding the oval lava-filled Crisium impact basin towards the bottom of the picture. Blue to orange: volcanic lava flows. To the left of Crisium, the dark blue Mare Tranquillitatis is richer in titanium than the green and orange maria above it. Thin mineral-rich soils associated with relatively recent impacts are represented by light blue colours. The youngest craters have prominent blue rays extending from them. The monochrome band on the right edge shows the unretouched surface of the Moon.
Image courtesy of NASA / JPL
Should humans go? Should robots go?
Or should both go? Humans can do more science than a robot in the same amount of time. Humans can learn quickly from their environment and apply their knowledge to assess a situation. However, a human mission costs a lot more money and endangers more lives than a robotic mission. Commonly, robotic missions are planned first to perform preliminary scientific studies: they use cameras and scientific instruments to study the area prior to human arrival, and deliver supplies in anticipation of later human missions. After a series of successful robotic missions, human missions are planned to perform more complex tasks, including advanced scientific experiments, habitat construction and exploration.
The unique benefits of human exploration are illustrated by the work of Apollo astronaut Jack Schmidt, the only geologist to have stood on the Moon. Jack Schmidt noticed a uniquely coloured orange rock on the lunar surface and he collected samples. These unplanned samples have proved to be extremely important to our understanding of the history of the Moonw4 and would have been missed completely if it were not for the training and experience of Jack Schmidt.
Should the experiments be done on the Moon or on Earth?
Lunar samples exist in an environment of minimal air and water and away from contamination sources such as humans, spacecraft and Earth’s atmosphere. Studying samples on the Moon therefore reduces the risk of sample change and contamination during transportation. However, the cost and technological challenge of developing and launching scientific instruments that are small enough and that function in lunar gravity limits what analysis can be performed on the lunar surface.
Therefore, samples that require multiple forms of analysis, or complicated instrumentation, should be tested on Earth. This implies that they must be carefully collected and transported to Earth to minimise changes and contamination. The rock boxes designed for the Apollo missions are a good example of how challenging this task can be. They were designed to keep oxygen and water away from the samples during the journey back to Earth; however, in some cases the abrasive nature of the lunar dust degraded the seals and the samples were exposed to air and humidity. Another consideration will be ice core sampling. Ice cores collected by drilling may be damaged by heat generated during the collection process, and collected ice cores will presumably need to be transported back to Earth in specially designed containers to protect the samples from heat, light, radiation, oxygen and biological contaminates.
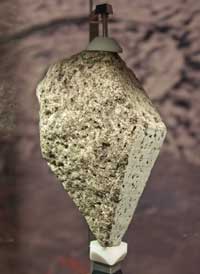
basalt was collected from the
Moon by the Apollo 15
mission. It was formed
around 3.3 billion years ago
and is now on display in the
National Museum of Natural
History in Washington, DC,
USA.
Image courtesy of Wknight94
/ Wikimedia
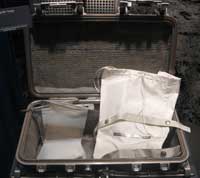
of lunar samples between the
Moon and Earth during the
Apollo programme. Inside the
case are several sample
collection and containment
bags, used on the surface for
initial sample collection and
sorting. These items are on
display at the National
Museum of Natural History
in Washington, DC, USA.
Image courtesy of Tyrol5
/ Wikimedia
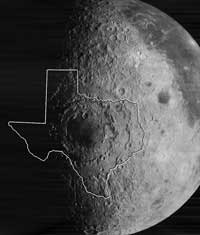
impact site approximately
the size of the American
state of Texas.
Image courtesy of Rick Kline,
Cornell University
How can samples be stored on Earth without compromising their quality?
The NASA curation facility in Houston, Texas, USA, has established successful procedures for handling soil samples under inert nitrogen gas, which ensures that they are well protected from humidity, biological and gas contamination. Dedicated facilities exist for the investigation of samples, and detailed records document what has been done with each sample. New samples, such as ice cores, will require additional method development to make sure that they are handled in a way that protects their scientific value.
How many missions to the Moon should be made?
Every mission should be built with the goal of furthering our knowledge and our exploration abilities. However, every mission requires a considerable investment in technology, human time and money. As an example, at its peak, 400 000 people worked on the Apollo programme, which cost US$20 billion in 1970w5 (equivalent to about US$120 billion in 2013 when adjusted for inflation). In contrast, the LCROSS / Lunar Reconnaissance Orbiter (LRO) mission cost US$583 million in 2009 (US$633 million in 2013 dollars)w6. The LCROSS / LRO mission was much cheaper than the Apollo programme, but its scientific value was much smaller and did not involve human exploration. When planning large exploration programmes, a balance must be achieved between robotic and human missions to maximise scientific return at a reasonable cost.
Who should pay? Who should go?
In the political and economic landscape today, very few countries have all the necessary resources and skills to launch their own lunar missions. International co-operation, where countries contribute skills and resources, allows all countries to contribute to a shared mission of long-term space exploration, to the Moon and beyond. It will be a challenge to agree which countries can send astronauts and equipment, and this could be an interesting topic to discuss with your students.
The benefits of returning to the Moon from a scientific and exploratory standpoint are enormous. However, many challenges must be overcome to accomplish those goals. This was achieved during the Apollo era, and there is a growing momentum within the scientific community today to do this again.
References
- Tranfield E (2014) Lunar diary: a chronicle of Earth’s journey through space and time, as seen from the Moon. Science in School 30: 36–43.
Web References
- w1 – NASA’s website on Lunar and Planetary Science has a map of the landing locations of the Apollo missions.
- w2 – To learn more about the Moon’s South Pole–Aitken Basin, see NASA’s website of the Solar System Exploration Research Virtual Institute.
- w3 – NASA’s LCROSS mission was designed to search for water on the Moon.
- w4 – To learn more about Troctolite 76535, the sample collected by Jack Schmidt during the Apollo 17 mission, see NASA’s Lunar Sample Compendium.
- w5 – The NASA History Program Office provides a detailed history of the Apollo programme.
- w6 – NASA’s extensive 2009 press kit for the LRO and LCROSS missions provides detailed information, including cost estimates.
Resources
- The website of the Lunar and Planetary Institute, which provides support services to NASA and the science community, includes information and resources for students and teachers.
- The tools used during the Apollo programme.
- A lecture series from 2008 about the Moon.
- NASA’s Human Spaceflight website provides details about Apollo programme, including its cost.
- Many different technologies have arisen from space exploration. For stories on these technologies and products, see NASA’s Spinoff publication.
- To learn more about how extraterrestrial materials are documented, preserved, prepared and distributed, visit the website of NASA’s Astromaterials Acquisition and Curation Office.
- To find out more about past and future lunar exploration, see:
- Baker A (2010) Space exploration: the return to the Moon. Science in School 16: 10–13
- Watch a video giving an overview of past, present and future Moon exploration.
Review
This article (part two of two) summarises challenges facing future journeys to our Moon.
The article stimulates questions around how or if scientific missions to the Moon provide sufficient benefit to mankind and around how these missions are carried out.
The students can consider questions like:
- Where should we land on the Moon, and should robots or humans be used?
- Should experiments be conducted on the Moon or Earth, and how should problems of transport be solved?
- How many missions should be planned, and who should pay?
Gerd Vogt, Higher Secondary School for Environment and Economics, Yspertal, Austria