Infectious cancers: the DNA story Understand article
What makes a cell turn cancerous – and how does a cancer become infectious? In the second of two articles on transmissible cancers, Elizabeth Murchison explains what the genetic details tell us.
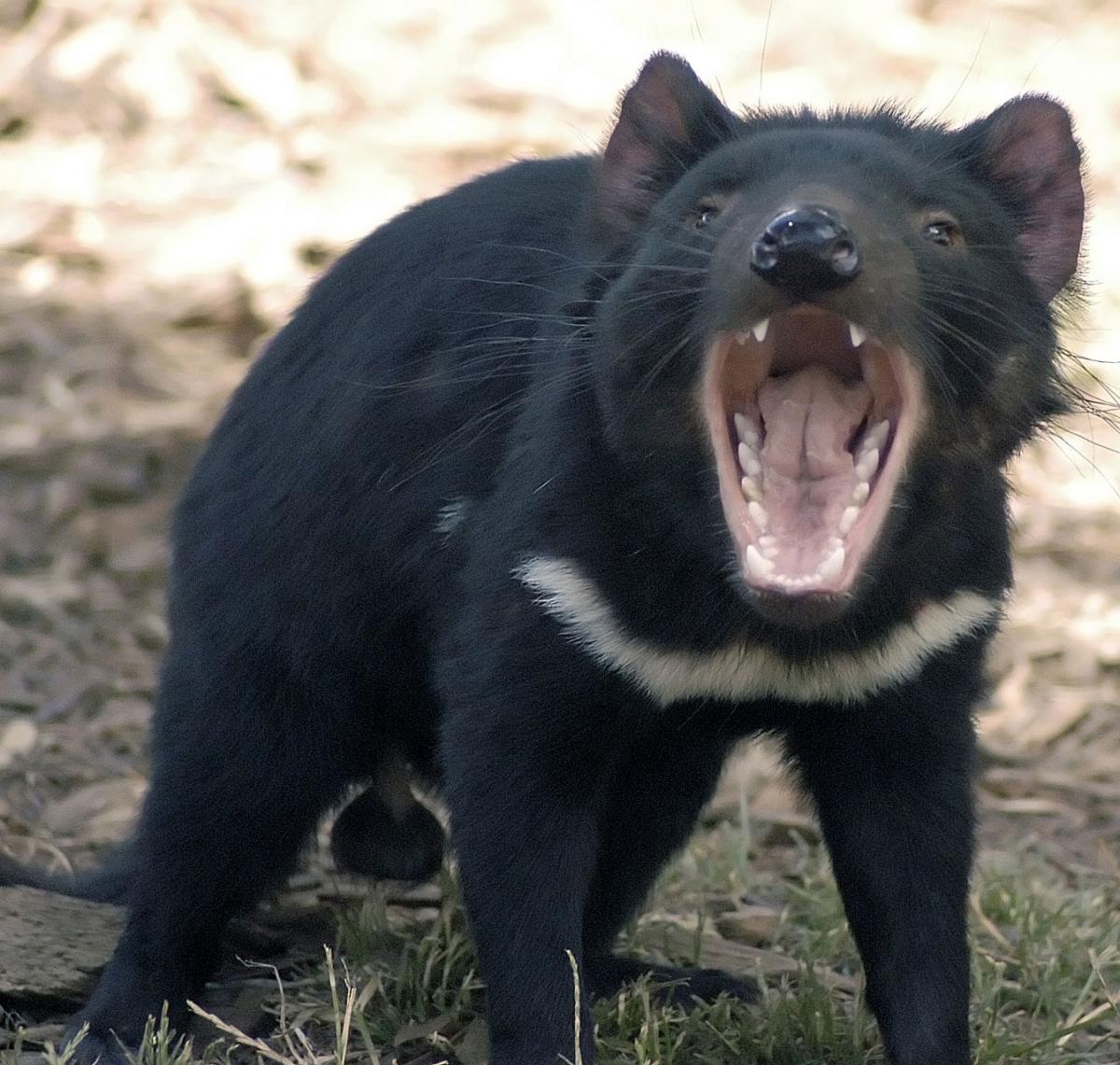
Image courtesy of Wayne
McLean/Wikipedia
Dr Elizabeth Murchison describes her work as ‘molecular archaeology’: instead of examining old stones and bones, she looks in minute detail at DNA molecules, trying to identify the key mutations that have altered the nature of the cells in which they reside.
Dr Murchison’s aim is to write the history of how a particularly nasty disease – devil facial tumour disease, or DFTD – has emerged and spread. This infectious disease is not caused by viruses or bacteria, but by tumour cells that have gained the ability to transfer from one individual to another, producing fatal tumours in each new host. Although it does not infect humans, DFTD is so virulent that although it emerged only in the past few decades, it is now threatening the species it affects – Tasmanian devils – with extinction.
Archaeological cancer
The nucleotide sequence of the DFTD tumour today is, in effect, an archaeological record made up of the genome of the original DFTD tumour from some 30 years ago plus the mutations that have accumulated since then. “As molecular archaeologists, we piece together the genome variation we find today, and try to work out which occurred in the original devil tumour, and which occurred after that,” says Dr Murchison.
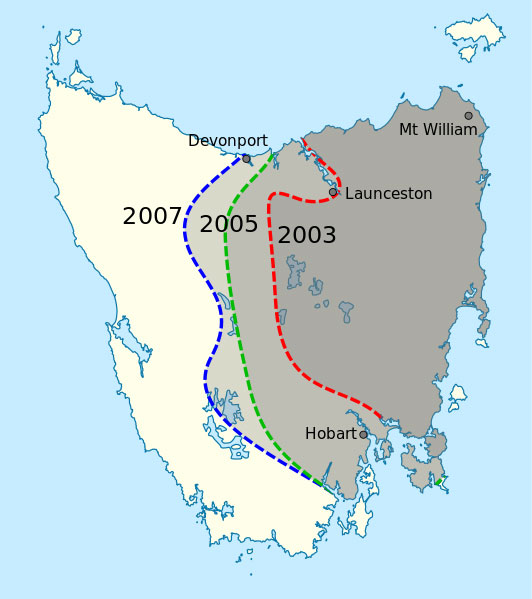
of DFTD in Tasmania in 2007.
Image in the public domain;
image source: Wikipedia
All cancers – not just transmissible ones – arise when a cell accumulates mutations. Every time a cell divides and its DNA is copied, there is a chance that the process will produce a new mutation. Other mutations occur as a result of carcinogens – for example, cigarette smoke or infection with the human papilloma virus (HPV). Some mutations that increase the risk of cancer can also be inherited, such as the BRCA1 gene, which is associated with breast cancer.
Typically, cells in human cancer tumours have undergone between 1000 and 5000 mutations in their DNA, although this number can increase to 100 000 or more when an individual has been exposed to carcinogens over a long period.
Surprisingly few mutations
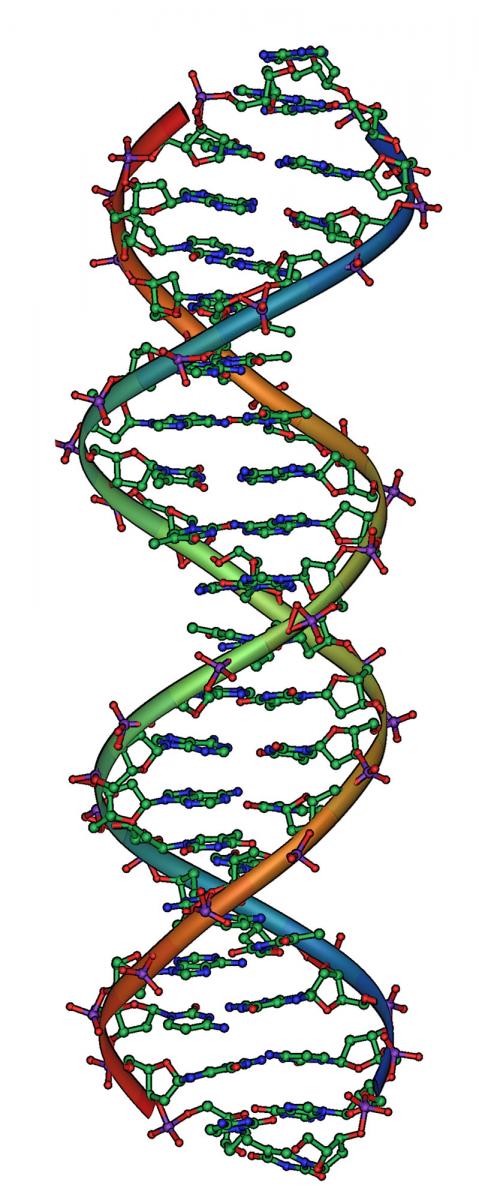
Initially, Dr Murchison’s team expected the number of mutations in the DFTD cancer to be much higher than in human cancers, because of the exceptional properties and rarity of transmissible cancers – but this is not the case. “We’ve estimated that DFTD has about 20 000 mutations, which is less than some human cancers,” she says. “This suggests that, to be transmissible, you don’t need enormous numbers of mutations – just the key mutations that allow the cancer to spread and survive in a new host.”
The team is now trying to pinpoint the identity of these key mutations. The first task was to work out the genetic information in normal Tasmanian devil cells, to act as a comparison with tumour DNA. “We had to start by sequencing the genome of Tasmanian devils, as there was no reference genome,” Dr Murchison says. “If we were to just sequence the genes from a DFTD cancer, we would have no way of knowing which genes were mutated and which were normal.” This was a challenge in itself, similar in scale to establishing the human reference genomew1.
The next step – comparing DNA from DFTD tumours to the reference genome – was also not as simple as it sounds. The disease first evolved in the 1980s, so when genetic variation is found between the reference genome and a particular DFTD tumour, it’s not clear whether the variation is derived from the original tumour or was picked up as a mutation over the past 30 years. To separate the original mutations that helped spawn the disease from the variations that occurred later, the team is now carrying out a ‘big screen’, sequencing DNA from hundreds of normal Tasmanian devils and comparing it to DNA from hundreds of DFTD tumours.
So far, the results have shown that the original transmissible tumour occurred in a female Tasmanian devil, because the tumour genome contains two distinct X chromosomes. The genetic basis for one key change has also been identified: the tumour’s ability to hide from its new host’s immune system by suppressing a gene that produces the ‘friend or foe’ molecular signal in normal cells. This finding, along with the fact that Tasmanian devils are genetically rather similar acro across the whole population, explains why cells from one individual are able to proliferate in another without triggering an immune response. The discovery is already being exploited in the search for a vaccine against DFTD. The research has also shed light on how the DFTD tumour cell line has evolved during its spread across Tasmania, revealing that there are several genetic subtypes of tumour.
Dr Murchison’s research is also looking at the only other known transmissible cancer – canine transmissible venereal tumour (CTVT), which occurs in dogs. Here, as with DFTD, her aim is to piece together genetic profiles of the animals in which the original tumours emerged and to trace their evolutionary path since then (see box).
Genetics of the canine transmissible venereal tumour
Genetic analysis has revealed that the canine transmissible tumours we observe today all have a common ancestor – which emerged 11 000 years ago! It also provides details of what the dog that had the first CTVT looked like, as shown in this sketch.
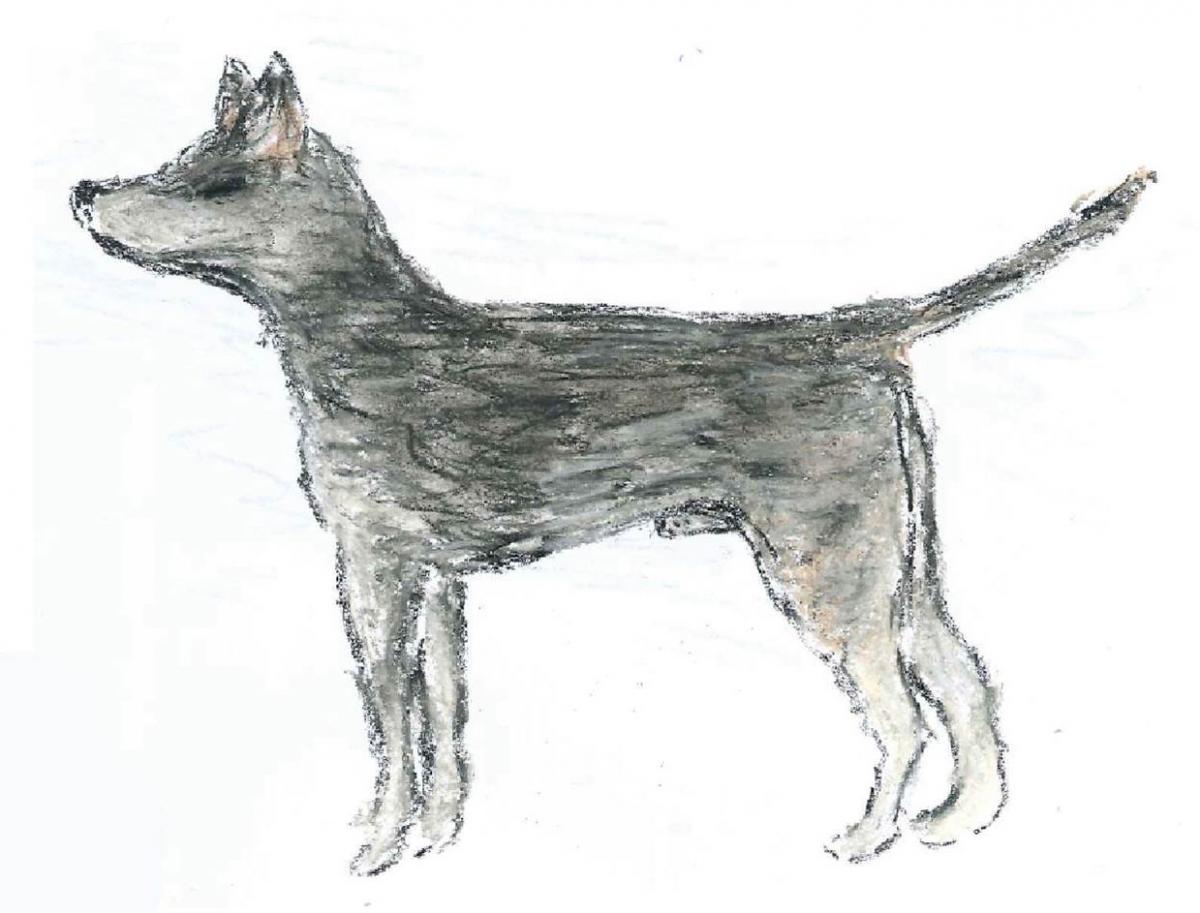
Image courtesy of Emma Werner
Gene name |
Associated physical characteristic |
---|---|
ASIP | ‘Agouti’ (mixed) coat colour |
CBD103 | Possible black coat colour |
KRT71 | Straight or wavy hair |
FGF5 | Short hair |
IGF1 | Medium or large size |
BMP3 | Probably pointy snout |
MGAM |
Adapted to starch and meat diet |
Fighting for survival
Back in Tasmania, people fighting for the conservation of Tasmanian devils are not relying solely on research efforts to deal with the threat of DFTD. A wildlife conservation project has started to establish a new population of healthy, uninfected Tasmanian devils on their own small island, separated from the main population. So while the scientific work to understand this devastating disease continues, the future for these fierce little animals may already be looking a little brighter. “We all have a strong common goal in trying to do something to help the devil,” says Dr Murchison.
Web References
- w1 – The human reference genome was determined by the Human Genome Project. For more information, visit the project website.
Resources
- For an introduction to DFTD, see the first of the two Science in School articles on the topic:
- Watt S (2015) Infectious cancers. Science in School 32: 6–9.
- Read more about DFTD and efforts to save the Tasmanian devil.
- Listen to a short lecture by Elizabeth Murchison explaining her work to a general audience.
- For an article on transmissible cancers, see:
- Giles C (2010) Sympathy for the devil. Wellcome News 62: 8–9
- This issue of Wellcome News can be downloaded from the Wellcome Trust website.
Review
The second part of the DFTD story is focused on the genetics of transmissible cancers. DFTD is a terrible disease that is reducing the Tasmanian devil population.
The article can be used to explore some interesting topics, such as ‘molecular archaeology’, DNA/genome sequencing, and the immunological aspects of normal and transmissible cancers. Perhaps an even more interesting idea is raised by the last paragraph of the text, which describes a conservation strategy for the Tasmanian devil. This could be used to develop a debate about the ethical concerns and boundaries of conservation efforts:
- Should humans interfere in a natural selection process?
Alternatively, should humans try to save a species that is threatened by natural causes?
- Thinking about the discoveries made by Charles Darwin about the Galapagos finches, do we have the right to select and separate a group of individuals from the main population? What consequences for a species are posed by the reproductive isolation of its populations?
Like the first article on this subject, this article stimulates biology teachers to deepen their knowledge of these topics.
Luis M. Aires, Antonio Gedeao Secondary School, Portugal