Inspired by nature: modern drugs Understand article
Many naturally occurring compounds are useful in medicine – but they can be fabulously expensive to obtain from their natural sources. New scientific methods of synthesis and production are overcoming this problem.
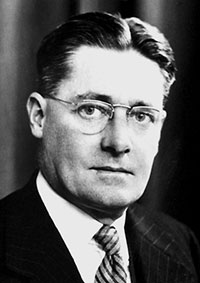
September 1898 – 21
February 1968) was an
Australian pharmacologist
and pathologist who shared
the Nobel Prize in Physiology
or Medicine in 1945 with Sir
Ernst Boris Chain and Sir
Alexander Fleming for his
role in the making of
penicillin.
Image courtesy of Nobel
Foundation/Wikimedia
Commons
The first patient ever treated with penicillin died one month later. The few grams of this antibiotic that were available at the beginning of 1941 were not sufficient to save the life of Albert Alexander, an English police officer who had been unlucky enough to get a bad infection from a scratch on his face. Although Alexander’s urine was processed to recover some of the used penicillin, this still did not produce enough. After a few hopeful days, Dr Howard Florey and his team were forced to admit an irrefutable fact: drugs are not truly useful unless there is an adequate supply.
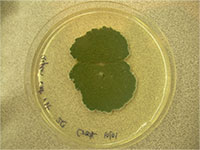
potato dextrose agar plate.
Image courtesy of
Ninjatacoshell / Wikimedia
Commons
Fortunately, the immense amount of scientific research carried out during the Second World War quickly remedied this situation and by 1943, an efficient method had been developed for cultivating large quantities of the Penicillium fungus and extracting the precious penicillin.
Drug development doesn’t always work like this, however. There are many potentially useful natural products that, even today, can be obtained only in minimal amounts from their natural sources. Plants, fungi and sessile marine organisms are particularly promising sources: unable to flee their predators, many of them specialise in chemical defence and this can be exploited to our advantage. One example is bryostatin, which is produced by Bugula neritina, a species of tiny marine invertebrates called bryozoans. Bryostatin could prove to be an effective treatment for oesophageal cancer – if it weren’t for the fact that it requires several tonnes of the animal to produce a few grams of the pure substance.
Natural compounds and modern medicines
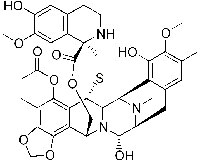
Chemical structure of
trabectedin.
Image courtesy of Edgar181/
Wikimedia Commons
People have used natural products medicinally since ancient times, and some four-fifths of the current world population still do so today. Although these products are traditionally used in the form of medicinal plants or fungi, improved versions of these drugs have more recently become available by isolating the active elements from the plant or fungal source. Since the first natural product ( morphine from the opium poppy, Papaver somniferum) was isolated in 1804, the use of pure compounds rather than crude plant or fungal preparations soon spread throughout the Western world.
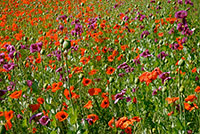
Image courtesy of
Nuuuuuuuuuuul / Flickr
In fact, the application of scientific knowledge and methods has dramatically increased the number of drugs of natural origin that are now at our disposal. By 1990, about 80% of drugs approved in the USA were either natural products or inspired by them (see Li & Vederas, 2009). There are hundreds of examples: antibiotics such as penicillin or erythromycin, anti-tumour drugs such as trabectedin and vinblastine, immunosuppressants such as cyclosporine and rapamycin that facilitate organ transplants, analgesics such as morphine and codeine, and antimalarials such as quinine and artemisinin.
These new drugs have become available via two main routes: clinical trials that have proved the effectiveness of some traditional remedies (for example, see Watt & Hayes, 2013); and the discovery of previously unknown, medicinally useful natural substances. Taken together, they have contributed to the success of modern medicine in extending our life expectancy from about 50 years at the beginning of the 20th century to the almost 80 years that it is today.
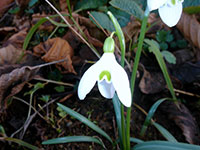
Galantamine is obtained
synthetically or from its
bulbs and flowers
Image courtesy of Karduelis/
Wikimedia Commons
Among all the sciences, chemistry stands out as having contributed perhaps most to this achievement. Chemical synthesis has made it possible to provide many drugs of natural origin in the dosage required for therapeutic use, despite the often very limited supply from their original sources. This is the case with galantamine, a compound produced by a rare flower from the Caucasus mountains that is proving to be one of the few substances capable of slowing the symptoms of Alzheimer’s disease. Despite its complex structure, this natural product is now produced commercially by synthesis from simple chemicals – a method that is much more affordable than its extraction from the Galanthus caucasicus flower itself.
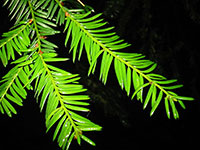
Image courtesy of Walter
Siegmund / Wikimedia
Commons
In addition, semi-synthetic processes – in which extracts from natural sources and chemical synthesis are combined – are now very common in the development of new drugs. One example of this is Taxol, used to treat patients with ovarian, breast and lung cancers or with advanced forms of Kaposi’s sarcoma. Originally isolated from the bark of the Pacific yew tree (Taxus brevifolia), clinical use of this source alone would have led to the tree’s extinction. As part of semisynthetic drug development, natural products are categorised into families on the basis of their chemical structure, with members of the same family often sharing many similarities.
This process revealed that the compound from the Pacific yew shared a similar structure with a much more accessible initial substance: 10-deacetylbaccatin III, found in the leaves of the European yew (Taxus baccata). A pathway to convert 10-deacetylbaccatin III to Taxol via just three simple chemical reactions was developed, providing an affordable and environmentally sustainable source of the drug (see box below)w1.
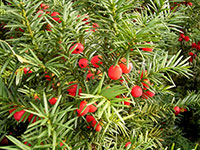
Image courtesy of Frank
Vincentz/Wikimedia Commons
Taking this a step further, we now often use natural products as molecular models for potential new drugs, rather than as the actual source or compound to be synthesised. In this strategy, a variety of synthetic compounds, or analogues, are produced with chemical structures that are similar to the original compound but easier to synthesise. The efficacy of each is then investigated, to identify compounds that are sufficiently simple to synthesise on an industrial level, and which also preserve the medicinal properties of the natural substance (see box below). This is being done in the case of bryostatin, and it is very probable that one of these analogues will form the biologically active part of a drug in the near future.
The semi-synthetic synthesis of Taxol
The extraction of Taxol (paclitaxel, figure 1) from the bark of the Pacific yew yields small amounts of the compound: 2000-2500 trees need to be felled to extract 1 kg of Taxol. The semi-synthetic synthesis of Taxol from 10-deacetylbaccatin III (figure 2), a related compound found in the foliage of the European yew, involves three simple chemical reactions (figure 3). Although 3000 kg of leaves from European yew are needed to obtain 1 kg of 10-deacetylbaccatin III, harvesting the leaves does not kill the treesw1.
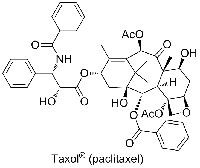
enlarge. The chemical
structure of Taxol
Images courtesy of David
Sucunza
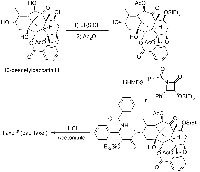
enlarge. The three-step
synthesis of Taxol from
10-deacetylbaccatin III
Images courtesy of David
Sucunza
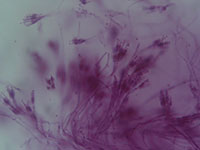
under the microscope)
Image courtesy of Peter Halasz
/ Wikimedia Commons
Bioreactors and beyond
Although chemical synthesis methods are often commercially competitive, another even more recent technique is gaining momentum: the artificial cultivation of cells from the natural product source. Growing cells in bioreactors to produce useful substances is now a widespread practice, and designing genetically modified organisms expressly for this purpose is swiftly becoming a more common reality (see box below).
The science of natural medicines continues to evolve. In the search for possible drugs, there are still thousands of plants, marine animals and micro-organisms left to study. This search continues alongside the hunt for new ways of obtaining useful products on a larger scale. After two centuries of intense scientific development, nature is no longer our limit, although it does continue to be our main source of inspiration.
Bioreactor synthesis to combat malaria
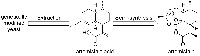
enlage. The extraction and
semi-synthetic synthesis of
artemisinin from genetically
modified yeast
Images courtesy of David
Sucunza
Malaria remains a major global health problem, killing more than half a million people each year. Currently, the most effective treatment is the natural product artemisinin, in combination with another drug (artemisinin combination treatments or ACTs). Artemisin in is produced by sweet wormwood (Artemisia annua) but this plant contains only a tiny fraction of artemisinin (between 0.001% and 0.8%). Supplies from sweet wormwood farms are limited, so ACTs cost US$1-2 per treatment course: too expensive for many patients in malaria-ridden countries.
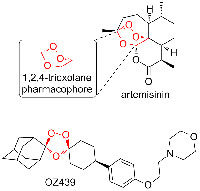
enlarge. The structure of
artemisinin and its synthetic
analogue OZ439
Images courtesy of David
Sucunza
In 2008, the pharmaceutical company Sanofi licensed a genetically modified yeast (Saccharomyces cerevisiae) to mass-produce artemisinic acid, a precursor of artemisinin, in bioreactorsw3,w4. By 2012, using this method (figure 4), the company has already produced almost 39 tonnes of artemisinic acid, the first industrial- scale deployment of synthetic biology for drug production. The stock could be converted to at least 40 million treatments. Although these treatments are not yet cheaper than the standard ACTs, researchers hope to make the fermentation process more efficient – and less expensive – in the near future.
However, ACT resistance has already been detected in South-East Asiaw5. As the antimalarial activity of artemisinin comes from its endoperoxide bridge (figure 5), several synthetic analogues based upon the 1,2,4-trioxolane pharmacophore, such as OZ439, are being studied as clinical development candidates.
References
- Li JWH, Vederas JC (2009) Drug discovery and natural products: end of an era or an endless frontier? Science 325(5937): 161-165. doi: 10.1126/science.1168243
- Watt S, Hayes E (2013) Monastic medicine: medieval herbalism meets modern science. Science in School 27: 38-44.
Web References
- w1 – Research in Review, published by Florida State University, tells the story of Taxol.
- w2 – The USA’s National Library of Medicine’s Drug Information Portal provides comprehensive details of Taxol (search for ‘paclitaxel’).
- w3 – Science Now describes the synthesis of artemisinin (Malaria drugmakers see the light).
- w4 – The website of Path, an international non-profit organisation focusing on global health, describes the organisation’s involvement in the development of semi-synthetic artemisinin.
- w5 – Nature Education’s Scitable website details the problems of ACT resistance (Artemisia annua: a vital partner in the global fight against malaria).
Resources
- The Plant Cultures website provides easy-to-read information about the roles that plants play in people’s lives all over the world.
- The Xplore Health website offers educational resources to teach about drug development.
- Based on one of the Xplore Health activities, one Science in School article explores the genetics of obesity: McLusky S, Malagrida R, Valverde L (2013) The genetics of obesity: a lab activity. Science in School 26: 25-30.
- Nicolaou KC, Montagnon T (2008) Molecules that Changed the World. Wiley-VCH: Weinheim, Germany
- Raviña Rubira E (2011) The Evolution of Drug Discovery: From Traditional Medicines to Modern Drugs. Wiley-VCH: Weinheim, Germany
- This book is freely available via Google Books.
- Le Couteur P, Burreson J (2003) Napoleon’s Buttons: How 17 Molecules Changed History. Jeremy P. Tarcher/ Putnam: New York, NY, USA
- This book can be freely downloaded from Scribd.
- A summarised version is available on the Napoleon’s Buttons website.
- Stuart DC (2004) Dangerous Garden: The Quest for Plants to Change Our Lives. Harvard University Press:Cambridge, MA, USA
Review
The article could be used in chemistry or biology lessons, particularly when teaching organic chemistry, ecology or conservation. For example, it could be used as the basis of a discussion about why natural products have been and still are so important for human health, and whether drugs developed in laboratories are always better than the remedies used by our ancestors. It could also be used as a starting point for a discussion about how chemistry, although often seen as an environmental threat, can in fact help to protect the environment.
Suitable comprehension questions include:
– How have natural products helped to preserve human health in the past?
– How are natural products helping to preserve human health today?
– How can chemistry help to protect endangered species?
– Why is it not possible to obtain all natural products we need from their natural sources?
Mireia Güell Serra, Spain