Cracking the mystery of how our planet formed Understand article
Studying the chemical composition of some of the planet’s oldest rocks has revolutionised our understanding of how our continents formed.
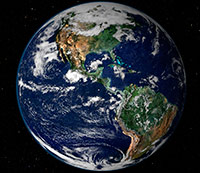
Nazmi El Saleous and Marit
Jentoft-Nilsen, NASA GSFC
Sometimes even the tiniest of rock fragments can hide big secrets. Our recent chemical analysis of African rocks has revealed that the continents we know today may have started to form more than a billion years earlier than was previously thought.
Our globe has changed over time
Earth formed about 4.6 billion years ago, from material in a giant molecular cloud called the solar nebula. Gravity caused this material to assemble into a sphere – Earth, with the densest forming the core, and the least dense forming the mantle. The crust and the upper part of the mantle – which together comprise the lithosphere – formed rigid plates, which move horizontally on top of the more malleable lower part of the mantle – the asthenosphere (figure 1).
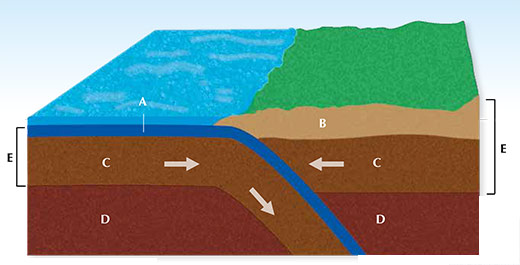
Plate tectonic movements can create subduction zones, where part of the lithosphere (E) descends into the asthenosphere (D). Subduction is a slow process that happens at high pressure (about 10 kilobar) and a temperature of less than 500 °C, and with a thermal gradient of less than 15 °C per kilometre.
Image courtesy of Nicola Graf
The organisation of these plates has changed dramatically over time (figure 2). About 2.5 to 4 billion years ago – during what is known as the Archaean eon – the lithosphere was partitioned into plates much smaller than the continents we know today. Later, during the Proterozoic eon, the plates joined together, forming one large supercontinent called Rodinia. Traditionally, this is believed to have been the situation 1 billion years ago. Subsequently, the continents started to drift away from these masses, progressively forming the globe we now recognise. The break-up of Rodinia is referred to as modern-style plate tectonics and is traditionally thought to have started around 900 million years ago.
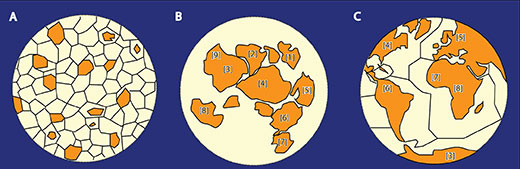
A) During the Archaean eon (4 to 2.5 billion years ago), the lithosphere was divided into many small plates.
B) About 1 billion years ago, during the Proterozoic eon, the plates are thought to have joined together, forming
one large continental mass: Rodinia. 1: Siberia; 2: Australia; 3: East Antarctica; 4: Laurentia; 5: Baltica;
6: Amazonia; 7: West Africa; 8: Congo craton; 9: India.
C) As the continents started to drift apart apart and join together again, they progressively formed the
globe we know today. This is traditionally thought to have started later in the Proterozoic eon,
around 900 million years ago.
Images courtesy of Hervé Martin (A, C), Kieff / Wikimedia Commons (B)
As this process occurs, plates collide. When one plate moves under the other and sinks into the mantle, it is called subduction (figure 1). Subduction is a slow process that happens at high pressure (about 10 kilobar) and a temperature of less than 500 °C, and with a thermal gradient of less than 15 °C per kilometre.
Testing the age of African rocks
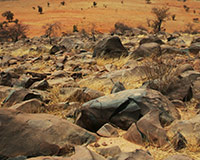
in the Essakane region of
north-east Burkina Faso. In
the foreground, you can see
weakly metamorphosed
volcanic rocks called basalts.
Image courtesy of Lenka
Baratoux
We didn’t set out to investigate plate tectonics, however. Instead, the purpose of our study was to use a new technique to learn more about the formation of metamorphic rocks about 2 billion years ago. We had not expected our work to have any implications for plate tectonics, which was generally thought to have started nearly 1 billion years later.
For the first stage of our study we visited several hundred geological sites around Africa (figure 3) and collected samples of greenstones. These rocks are known to have undergone metamorphosis – a change from one rock type to another – about 2 billion years ago. Based on previous knowledge about the metamorphism of rocks during this period, it was thought that they must have formed under conditions of low pressure (no more than 5 kbar) and temperatures ranging from 200 to 700 °C.
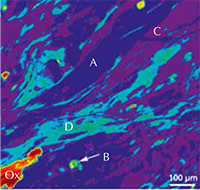
of a complex metamorphic
rock containing quartz (A),
garnet (B), phengite (C),
chlorite (D) and iron oxides
(Ox). The image was obtained
at ESRF using very intense
X-ray beams.
Image courtesy of Vincent de
Andrade
Next, we investigated the composition of minerals in these rock samples using microprobe analysis. This is a range of techniques that includes microscopy and back-scattered electron imaging, which distinguishes heavy elements, which scatter electrons well, from light elements that do not. We also performed chemical mapping, which shows where particular minerals are found in the samples.
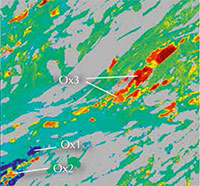
chemical mapping showing
three types of iron oxides:
Ox1, which is low in Fe3+;
Ox3, which is rich in Fe3+;
and Ox2, which has
intermediate levels of Fe3+.
The image was obtained at
ESRF using very intense
X-ray beams.
Image courtesy of Vincent de
Andrade
Furthermore, we carried out experiments at the European Synchrotron Radiation Facility (ESRF; see box) to decipher the very fine chemical structure of some of our samples. Synchrotron X-ray beams are billions of times brighter than the beams produced by a hospital X-ray machine, allowing them to resolve the structure of matter at a level of detail impossible to reach with standard X-rays.
Using very thin slices of rock, we were able to map their chemical composition. We found that they contained quartz, garnet, phengite, chlorite and iron oxides (figures 4 and 5). But what did this tell us about how the rocks formed and under what conditions?
To interpret our results, we used computer calculations based on different chemical parameters that we measured. For example, we analysed the ratio of H2O to CO2 in the fluids trapped within the quartz, and measured the ratio of Fe3+ to Fe2+ present in the rocks (figure 5). There are many different chlorites (e.g. magnesium chlorite, iron chlorite) and several different forms of phengite (which may contain, for example, magnesium or iron). The precise chlorites and phengites that we observe in the metamorphic rocks depend on the conditions at the time of rock formation. These are the H2O:CO2 and Fe3+:Fe2+ ratios as well as on the pressure and temperature. Measuring the ratios of these different chemicals in our rock samples therefore allows us to work backwards to calculate exactly the temperature and pressure conditions under which the rocks formed.
Revolutionising our understanding of plate tectonics
Using these calculations, we demonstrated that the chlorite and phengite composition in the rocks of western Africa was obtained under high pressure (about 10 kbar) and a low temperature of less than 500 °C. This was surprising, because these pressure and temperature conditions are found only in subduction zones. Since the rocks we studied date back more than 2 billion years, our results imply that modern-style plate tectonics existed 2 billion years ago, far earlier than the 900 million years ago that scientists had previously thought.
Our discovery has changed the scientific understanding of the geodynamics of Earth. So when, then, did modern-style plate tectonics actually begin? And how widespread were these gigantic land movements? To address these questions, our next step will be to study other rocks of the same age and older. In particular, we plan to visit Yilgarn Craton in Australia and the Barberton area in South Africa, to examine their chlorite- and phengite-containing metamorphic rocks.
More about ESRF
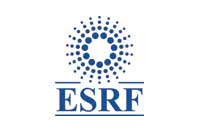
The European Synchrotron Radiation Facility (ESRFw1) is one of the most intense sources of X-rays in the world. Thousands of scientists come every year to ESRF to carry out experiments in materials science, biology, medicine, physics, chemistry, palaeontology and cultural heritage. ESRF is a member of EIROforumw2, the publisher of Science in School.
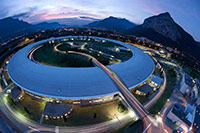
French Alps, the European
Synchrotron Radiation
Facility uses brilliant beams
of X-rays to resolve the
structure of matter. A
synchrotron is a type of
cyclic particle accelerator: at
ESRF, the synchrotron light
travels at great velocity
around the giant grey ring.
Image courtesy of Ginter / ESRF
Acknowledgement
The authors would like to acknowledge the help of Dominique Cornuéjols, from ESRF’s communications department, in preparing and translating material for this article.
Web References
- w1 – Learn more about ESRF.
- w2 – EIROforum is a collaboration of eight of Europe’s largest international governmental scientific research organisations, which combine their resources, facilities and expertise to support European science in reaching its full potential. As part of its education and outreach activities, EIROforum publishes Science in School.
Resources
- The Exploring Earth website offers a useful interactive animation of the rock cycle.
Institutions
Review
This article about two scientists’ discoveries about plate tectonics offers a taste of cutting-edge research. In a clear and concise style, the authors guide their readers from the basics of plate tectonics theory to the object of their research, focusing on its implications for Earth’s history and on the new questions raised by this breakthrough.
As an earth science teacher I find the article interesting for different reasons:
- It focuses on a period of Earth’s history that is rarely addressed in school textbooks.
- It gives an interesting view on early plate tectonics.
- It provides details of the methodology and equipment used in the research.
- It is a vivid example of the scientific method.
I recommend this article to secondary-school teachers keen to interest their students in earth science and scientific research in general. It could provide valuable background reading to raise students’ interest before addressing some topics usually considered tedious, such as minerals and plate tectonics.
The text offers multiple links not only to earth science topics (Earth’s history, plate tectonics, mineralogy, the rock cycle, geochemistry, investigative techniques, Africa, greenstone belts and gold mining), but also to chemistry (iron oxides and redox reactions) and physics (X-rays and synchrotron radiation machines, scanning electron microscope, pressure, temperature and phase transitions). In addition, it is a good case history for discussing the scientific method.
Given the language in which it is written, the article could easily be used for comprehension exercises, such as:
- The minerals studied formed under conditions of:
- High pressure (10 kbar) and low temperature (less than 500 °C)
- Low pressure (5 kbar) and high temperature (more than 700 °C)
- Low pressure (5 kbar) and temperatures ranging from 200 to 700 °C
- High pressure (10 kbar) and temperatures ranging from 200 to 700 °C.
- The composition of the different types of chlorite and phengite depends on:
- The ratio of H2O to CO2
- The ratio of Fe2+ to Fe3+
- The temperature and pressure of formation
- All of the above.
Giulia Realdon, Italy