Birds on the run: what makes ostriches so fast? Understand article
What makes ostriches such fast runners? Nina Schaller has spent nearly a decade investigating.
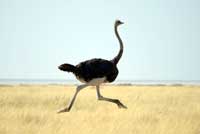
Carnemolla / iStockphoto
When admiring a soaring seagull or a diving penguin, we rarely consider that these feathered animals have something very rare in common with us: whereas most other animals move on four, six or more legs, birds and humans are the only true bipeds.
Evolution has solved the challenge of moving on two legs in two ways: humans are plantigrade (we place our entire foot on the ground when we walk or run), whereas birds are digitigrade (they walk on their toes, or digits).
Some avian species can run more quickly not only than humans run, but even faster than their flying counterparts fly. The fastest long-distance runner is the African ostrich (Struthio camelus). At a steady 60 km/h with top speeds exceeding 70 km/h, it could cover the 42 km Olympic marathon in 40 minutes rather than the two hours needed by a human. This remarkable combination of speed and endurance allows the ostrich to cover great distances to find fresh grazing pasture or to outdistance hungry hyenas.
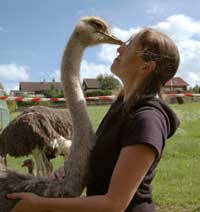
grown co-workers, Tiffy
Image courtesy of Gisela Löffler
for Bild der Wissenschaft
Scientists have long explored the challenges of terrestrial locomotion, particularly the running abilities of dogs and racehorses. However, studies on avian locomotor modes have typically explored flight dynamics while paying less attention to cursorial species (those that are specialised for running).
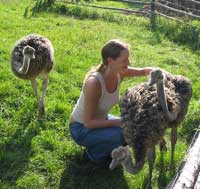
in their new home
Image courtesy of Jürgen Gass
After finishing my degree in biology in 2002, I volunteered at Frankfurt Zoo in Germany, where I became fascinated by the ostrich’s racing ability and decided to investigate it. The hypothesis of my PhD research was that the ostrich locomotor system transmits power to the ground with a high degree of efficiency, maximising energetic output (speed and endurance) while minimising energy demands (muscular and metabolic work).
To test my idea, I decided to study both form and function of the ostrich locomotor system. Using dissection, I explored ostrich anatomy, searching for specialised limb structures that might reduce the metabolic cost of locomotion. Simultaneously, I studied the biomechanics of live ostriches: how physical forces acted on their anatomy when they moved.
To enable close observation of natural motion sequences, I hand-raised three ostriches in a large outdoor enclosure and, over four years, habituated them both to me and their experimental racetrack. Mutual trust was crucial: a kick from an ostrich can kill a lion.
Maximising speed: long, light legs
In a running animal, higher speeds are achieved by increasing both the length and frequency of steps. Longer legs can swing further, and if the leg’s muscle mass is located proximally (close to the body), the leg can then swing faster, in the same way that moving the adjustable weight of a metronome closer to the pivot increases the tempo.
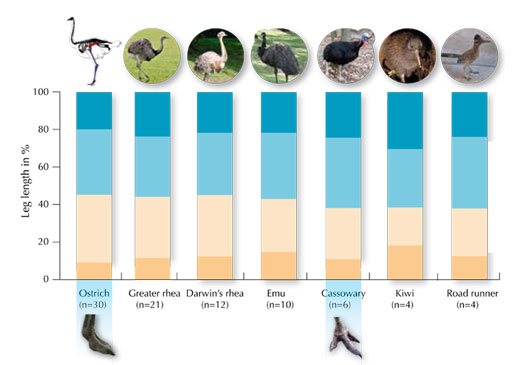
Swinging portion of the leg:
Thigh bone (held horizontally): dark blue
Shin bone: pale blue
Tarsometatarsus: pale orange
Main toe: dark orange.
Click on image to enlarge
Image courtesy of Nina Schaller
Greater rhea, Rhea americana
Image courtesy of Quartl; image source: Wikimedia Commons
Darwin’s rhea, Pterocnemia pennata
Image courtesy of Jennifer Bergk; image source: Wikimedia Commons
Emu, Dromaius novaehollandiae
Image courtesy of Quartl; image source: Wikimedia Commons
Cassowary, Casuarius casuarius
Image courtesy of Dezidor; image source: Wikimedia Commons
Kiwi, Apteryx mantelli
Image courtesy of the Maungatautari Ecological Island Trust; image source: Wikimedia Commons
The greater roadrunner, Geococcyx californianus
Image courtesy of Back1a5h; image source: Wikimedia Commons
Cassowary feet
Image courtesy of Bjørn Christian Tørrissen; image source: Wikimedia Commons
To investigate this principle, I compared leg segment lengths (Figure 1) and muscle mass distribution of fast-running, ground-dwelling bird species. Of all cursorial birds, the ostrich possesses the longest legs relative to its size and has the longest step length when running: 5 m. In addition, to a greater degree than other bird species, it has the majority of its leg musculature located very high on the thigh bone and hip, whereas the lower swinging elements of its leg are comparatively light, moved by long, mass-reducing tendons (Figure 2). This arrangement optimises the ostrich leg for high-velocity locomotion, giving it both a long step length and a high step frequency.
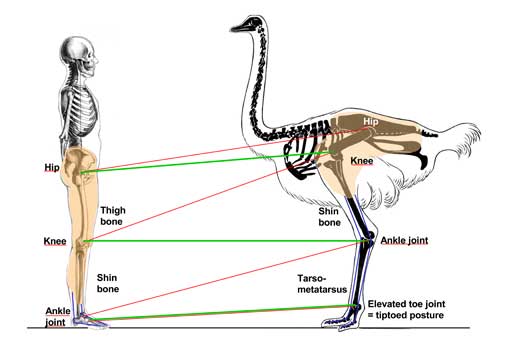
In the ostrich, muscle mass is concentrated at the top of the leg, while long tendons prevail in the lower regions. Shaded areas show major muscle mass distribution, blue lines indicate location of major tendons. Click on image to enlarge
Image courtesy of Nina Schaller
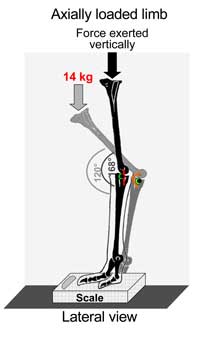
showing the ability of the
ankle joint ligaments to
passively support weight
from above (only the left
side of the left leg is shown).
The ankle joint has
contoured bone protrusions
(in green) on either side.
When the joint is fully
extended (168°), the
ligaments on either side (in
red) are tensed because they
pass over this protrusion,
thereby stabilising the joint.
When the ostrich lifts its toes
off the ground, the ankle
joint is flexed below 140° and
the ligaments (in orange) are
free to slide around the
protrusions: the stabilising
mechanism is relaxed. Click
on image to enlarge
Image courtesy of Nina Schaller
Maximising endurance: stable joints
A wide range of joint motion allows humans to climb trees or ballet dance, but this flexibility has a cost. When we run, muscle power is used for propulsion but also to prevent sideways joint movement, thereby increasing our energy requirements over a given distance. I suspected that ostriches had a more efficient approach.
Unlike energy-consuming muscles and their tendons, ligaments can act as a ‘joint corset’, limiting sideways motion without consuming energy. To demonstrate that this mechanism was present, I filmed my running ostriches from various angles to record the range of motion of their legs. I then repeated these measurements with an intact dead ostrich, and finally with a dissected ostrich leg that had had all muscles and tendons removed, leaving only the skeleton and joint ligaments. The range of sideways motion in live and dead ostrich specimens was nearly identical. In contrast, a similar comparison in humans would reveal a huge difference in sideways motion range, especially at the hip joint, which is stabilised by muscle action. My measurements showed that ligaments are the main elements that guide an ostrich leg through the stride, allowing muscle power to be devoted almost exclusively to forward propulsion.
When manipulating the dissected ostrich legs, I made a further new discovery. When trying to flex the ankle joint, I had to overcome some resistance – an unexpected finding in a lifeless limb devoid of muscles. When I released the joint, it snapped back to an extended position, suggesting that ligaments were passively keeping the bird’s leg extended. To test this theory, I exerted pressure from above on the standing, dissected leg until the ankle joint collapsed into a flexed position (Figure 3). It required 14 kg of downward force — 28 kg of weight that an ostrich standing on two legs would not be required to actively support when walking or running. This experiment showed that saving metabolic energy by using ligaments as a passive leg-stabilising mechanism is an excellent locomotor endurance strategy.
Making ground contact
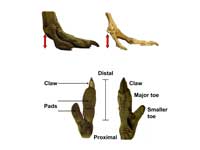
ostrich. Left to right: average
standing position with lateral
smaller ‘outrigger’ toe; toe
skeleton (red arrows indicate
elevated toe joint); the ‘foot’
viewed from above; the ‘foot’
viewed from below.
Distal = away from the
body; proximal = closer to
the body. Click on image to
enlarge
Image courtesy of Nina Schaller
We have seen that light limbs are a precondition for fast, efficient locomotion and that one way in which the ostrich achieves this is by concentrating the leg muscle mass close to the hip joint. A further strategy for reducing lower-leg mass involves specialised toe morphology and positioning. This can also be observed in other cursorial animals; modern horses, for example, have evolved from five-toed ancestors to gallop on the toenail of their middle toe – the hoof. The ostrich has undergone a similar evolution: whereas most birds have four toes and the majority of large flightless birds possess only three, the ostrich is unique among birds in walking on only two toes (Figure 1). Furthermore, it is the only bird to walk on the tips of its toes.
I wondered how this, the largest and heaviest living bird, manages to balance and grip at high-speed on tiptoe. Since there is no established method for investigating toe function in live birds, I used a pressure plate, commonly used by orthopaedists to analyse pressure distribution in human feet. I trained my ostriches to walk and run over the plate, capturing high-resolution real-time data of ostrich ‘foot’ pressure during ground contact. This showed that the big toe supports the majority of the body mass while the smaller toe prevents the ostrich from losing its balance by acting as an outrigger, especially during slow walking.
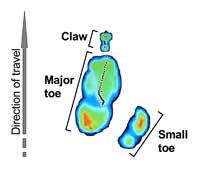
profile of an ostrich’s right
‘foot’ recorded with a
pressure plate. Areas in red
indicate very high load; dark
blue indicates minor load.
Click on image to enlarge
Image courtesy of Nina Schaller
At high speeds, the toes’ soft soles dampen impact stresses, while the spring-loaded tiptoed posture acts as an additional shock absorber (red arrows in Figure 4). The claw barely contacts the ground during walking, but exerts pressures of up to 40 kg/cm² when the bird runs. The claw penetrates the ground like a hammered spike to ensure reliable grip at 70 km/h – maximum speed with minimal energy, ideal for endurance running on the level ground of the African savannah (Figure 5).
Practical applications
My research has gone a long way to improve our understanding of how the ostrich runs so fast for so long. Now that we understand these biomechanical strategies, perfected over 60 million years of evolution, we may be able to adapt them in modern technologies such as bipedal robotics, suspension systems, and joint-stabilisation engineering. Already, some of my findings have inspired developers of ‘intelligent’ human prostheses to adapt features of ostrich legs and toes, which may allow amputees a wider range of function and manoeuvrability.
Resources
- The ‘Confessions of an ostrich’ give the ostrich’s point of view, and include links to other resources. See: http://tolweb.org/treehouses/?treehouse_id=3303
- This introductory lesson on ostriches consists of an essay and a quiz for the students. It is suitable for lower secondary-school level. See: www.lessonsnips.com/lesson/ostriches
- A similar activity for primary-school students can be found here: www.enchantedlearning.com/subjects/birds/printouts/Ostrichquiz.shtml
- The National Geographic Kids website has a multimedia ‘Creature Features’ page on ostriches. See: http://kids.nationalgeographic.com/kids/animals/creaturefeature/ostrich
- For an introduction to the biomechanics of walking, see: www.pt.ntu.edu.tw/hmchai/BM03/BMClinic/Walk.htm
- The Society for Integrative and Comparative Biology’s activities on bone and joint biomechanics were developed for university students, but can easily be adapted for older secondary-school students. To build models of joints, see: www.sicb.org/dl/biomechanicsdetails.php3?id=19
- For a slide show overview of human locomotion and its biomechanics, with links to sport, see: http://tinyurl.com/c2yrxca
- To download the slide show, you will need a Google email account.
Review
The surprising yet obvious statement that ‘birds and humans are the only true bipeds’ introduces the account of the research that Nina Schaller has been doing for nearly a decade. She offers us a picture of the multidisciplinary approach to a complex phenomenon – the outstanding speed and endurance of the ostrich – investigating the biomechanics and efficiency of the bird’s performance by means of anatomy (dissection) and physiology (functional study). The listed resources, in addition, provide a wealth of information and teaching materials on ostrich and human locomotion.
The article would be an interesting and useful means to address topics in biology (biomechanics – bones, muscles, tendons and ligaments; evolution – homology and analogy) and physics (efficiency, forces, speed, springs and motion) at both upper- and lower-secondary school. For example, it could be used to address the biomechanics of walking and running in different species, the evolution of bipedal locomotion in birds and humans, and the functional aspects of lower limb prostheses (for instance those used by the sprint runner Oscar Pistorius). It could also provide valuable background reading before a visit to a natural history museum or zoo, or to a robotics lab.
Suitable comprehension questions include:
- The hypothesis of Nina Schaller’s research was that the ostrich’s locomotor system:
- maximises energetic output and energy demand
- minimises energetic output and maximises energy demand
- maximises energetic output and minimises energy demand
- minimises energetic output and energy demand.
- Ostrich legs have
- musculature located high on the thigh bone and short tendons
- musculature located high on the thigh bone and long tendons
- musculature located low on the thigh bone and short tendons
- musculature located low on the thigh bone and long tendons.
Giulia Realdon, Italy