Chemistry and light Understand article
Peter Douglas and Mike Garley investigate how chemistry and light interact in many aspects of our everyday life.
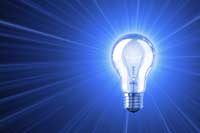
/ iStockphoto
Since time immemorial we have used the Sun’s rays to warm ourselves during the day and the glow of a flame to light up our world at night. Today, we control the inter-conversion of energy to make light from electricity, heat and chemical reactions; in our daily lives, we use chemistry and light in communication, electronics, medicine and entertainment; and photochemists are working for a cleaner, brighter future by devising new methods to convert sunlight into useful energy and to remove pollutants photochemically.
Making light: lamps, lasers, LEDs and liquid light
The essential component in the process of making light is the inter-conversion of energy. Different types of lamps and lighting devices convert energy in different ways and with differing efficiencies.
In the tungsten lamp, electrical energy heats a filament to a white-hot glow; thus, thermal energy is converted into light energy. Light emission from the solid filament is a continuum and gives a visible spectrum, much like a rainbow. Unfortunately the efficiency with which electrical energy is converted to visible light energy is only about 5-10%.
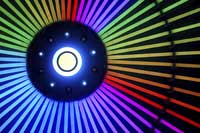
Image courtesy of mipokcik
/ iStockphoto
In the fluorescent lamp, electrical energy is converted into atomic excitation energy in the vapour of mercury atoms inside a tube. In this case, the energy conversion efficiency is about twice that of a tungsten bulb at around 20%. However, the spectrum of light emitted by these lamps (when the electrons return to their non-excited state) is not continuous; instead, light is emitted at specific wavelengths and colours corresponding to the electrical energy levels of the mercury atoms. Household fluorescent tubes, therefore, have a coating of white phosphor inside to convert this light emission into a more continuous spectrum. Replacing the mercury vapour with other gases, such as neon (which gives an orange light) or other inert gases, allows the production of many different coloured fluorescent lamps, used for luminous displays and signs (see image). Different phosphor coatings also change the colour of the light from the lamp.
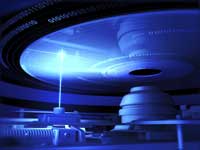
Image courtesy of Petrovich9
/ iStockphoto
Electrical energy can be converted still more efficiently into light by light-emitting diodes (LEDs); electricity excites electrons in specially designed semiconductor (see glossary for all italicised terms) layered structures to produce visible light, with a conversion efficiency of up to 35%. Electroluminescent paper uses the same principle. Many lasers also use electrical energy to generate high-intensity laser light, which can be collected into a very narrow, intense beam. High-power lasers can cut metal or can even be used as light scalpels in surgery.
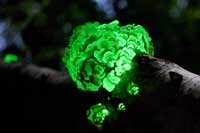
stipticus displaying
chemiluminescence
Public domain image; image
source: Wikimedia Commons
Lasers are also used in communications and digital technology in barcode readers and optical disc readers.
‘Liquid light’ relies on a different kind of energy conversion – chemiluminescence – to produce a cold light through chemical reactions rather than thermal energy. This is how chemiluminescent light sticks work. In nature, glow worms and creatures that live in the darkness of caves or the deep sea use chemiluminescence for inter- and intra-species signalling.
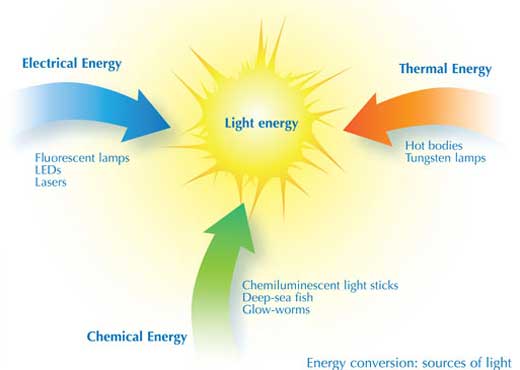
Image courtesy of Nicola Graf
Photochemistry: fluorescence, plastics, photography and medicine
One type of light emission – fluorescence – is used in optical brighteners in washing powders. They absorb the small amount of invisible UV light in the Sun’s spectrum, and re-emit it as blue light, making clothes look ‘whiter than white’. Fluorescence is also used in the security markings on banknotes, while phosphorescence (similar to fluorescence but with a longer life) is used in safety signs. Fluorescence, phosphorescence and chemiluminescence are also used in novelty items such as luminous body paint, hair gel, lipstick and jewellery.
Light can be used to make plastics. Plastics are made by polymerisation – the joining together of lots of small molecules (monomers) to give one long molecule (a polymer). This process often needs something with enough energy to start the process going, although once started, the energy released in the joining-up step is usually enough to keep the process going. When light is absorbed by a molecule, that molecule becomes more energetic because of the energy of the photon it has absorbed; we say the molecule has been excited. These excited-state molecules are excellent polymerisation initiators because of this extra energy – thus light can be used to convert liquid monomers into a solid plastic. This process called photopolymerisation gives us dental glues that harden under UV light (see image below), as well as photo-curing paints which are used to print images onto a wide variety of substrates – soft-drink cans for example. Unlike conventional paints, a photo-curing paint is set by light rather than by heat and/or air oxidation.
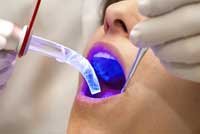
in dentistry
Image courtesy of dem10 /
iStockphoto
Photographic film relies on the photochemical properties of silver halides. When a grain of silver halide on a film absorbs a photon of light, an atom of silver is made. The film is then chemically processed, and the metallic silver grains comprise the black part of a black and white negative.
The best known use of photochemistry in medicine is the treatment of jaundice, a build-up in the body of a yellow, fat-soluble, neurotoxic substance called bilirubin.
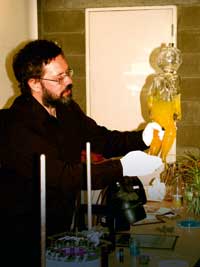
baby ‘Bili Rubin’, show how
light is used in the cure for
jaundice
Image courtesy of Peter Douglas
Bilirubin is generated constantly as a by-product of haemolysis (the breakdown of red blood cells), but it is normally metabolised in the liver into a water-soluble form that is subsequently excreted. If, however, the liver is damaged or not fully formed, the uncontrolled build-up of bilirubin can be fatal. Exposure to blue light cures jaundice through a photochemical transformation that converts bilirubin into a water-soluble form, allowing its excretion. Hospital wards for premature babies – who are particularly prone to jaundice – have cots with special lights for the treatment of jaundice.
Another medical application for photochemistry is the use of photodynamic therapy to fight cancer. A highly coloured compound with a particular photochemistry is injected directly into a tumour. This compound preferentially adsorbs to cancer cells rather than to normal cells and, when irradiated with light from a laser or other source, forms excited-state molecules that react with oxygen to generate chemicals that are lethal to cancer cells.
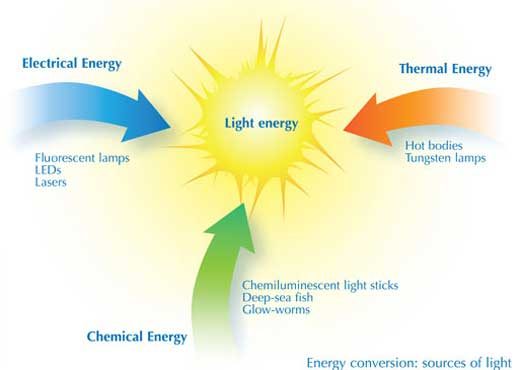
Image courtesy of narvikk / iStockphoto
Clean energy, clean planet
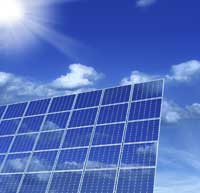
Image courtesy of narvikk /
iStockphoto
The world’s energy demands are increasing whereas its non-renewable supplies are limited. One way to address this problem is by using less energy (e.g. with energy-saving light bulbs). Another way is to exploit renewable sources of energy such as the silicon solar cell, which converts light energy into electricity. The original single-crystal cells, developed in the 1950s to power satellites, were very expensive. Now, much cheaper polycrystalline and amorphous silicon cells are commonly used in powering items such as calculators and battery chargers, as well as in larger-scale domestic, industrial and even national energy installations.
In the classroom, the conversion of sunlight into electricity can be observed in a Grätzel cell, which employs artificial photosynthesis using natural dyes found, for example, in cherries (see Shallcross et al., 2009).
Another real problem for the future is the need for a clean transportable fuel. Hydrogen can be generated indirectly from solar energy through the electrolysis of water, but this reaction is wasteful in energy terms. Thus, a great deal of research currently focuses on splitting water into hydrogen and oxygen directly using sunlight.
How else can chemistry and light contribute to a clean planet? Titanium dioxide (TiO2) is a semiconductor with some interesting photochemical properties. It is used as a white pigment because it scatters visible light effectively. It also absorbs UV light. When used as pigment or as a UV absorber in sunscreens, each particle is coated with silica to prevent the bare TiO2 surface from coming into contact with its environment. This is necessary because when irradiated with UV light, TiO2 particles generate very reactive chemical species which will destroy any complex molecules next to them. This apparent disadvantage of bare TiO2 is now put to great use in the destruction of pollutants because when bare titanium dioxide is irradiated with UV light in solution, it completely destroys any organic compounds close to its surface. As a result, any organic pollutants present are completely mineralised to carbon dioxide, water, and ammonium and chloride ions.
These examples illustrate that chemistry and light are all around us – they are an important part of our technological world, and have an important role in our cleaner, brighter future!
Glossary
Chemiluminescence: The generation of light directly from a chemical reaction, e.g. the light from glow-worms, and chemiluminescent light sticks.
Electroluminescence: The direct generation of light from electricity, e.g. in the display screens of mobile phones.
Fluorescence: The emission of light associated with the transition of an electron from an excited state to a lower-energy state during which an electron does not have to change its spin. Because of this, the light emission happens very quickly after excitation, usually within a few nanoseconds. Thus if the excitation source is removed, fluorescence stops within a few nanoseconds.
Luminescence: A general term for the emission of light.
Phosphorescence: The emission of light associated with the transition from an excited state to a state of lower energy where an electron does have to change its spin. This allows the light emission to occur more slowly, sometimes over a timescale of seconds in molecules and often longer in solid-state phosphors. Thus if the excitation source is removed, phosphorescence can sometimes still be seen, e.g. ‘glow in the dark’ stickers or the afterglow from the phosphor on a fluorescent tube which can be seen immediately after the light has been switched off (i.e. once the fluorescence has stopped).
Photodynamic therapy: A medical procedure in which light is used to destroy tumours in cancer treatment.
Photopolymerisation: The process by which light is used to create a polymer from monomeric molecules. Photopolymerisation is used to set photo-curing paints, inks and glues. Photo-curing glues are commonly used in dentistry.
Semiconductor: For the purposes of this article, the important feature of a semiconductor is the arrangement of electronic energy levels into two ‘bands’: a full low-energy ‘valence band’ and an empty high-energy ‘conduction band’. Excitation promotes an electron from the valence band into the conduction band, leaving behind a ‘hole’. Both the hole and the electron are mobile and can migrate to the semiconductor surface where they can act as oxidant and reductant, respectively, in the destruction of pollutants.
The London International Youth Science Forum
The basis of this article was a demonstration lecture by Dr Peter Douglas and Dr Mike Garley on the use of chemistry and light in our everyday lives. The lecture was part of the London International Youth Science Forum 2009 and was attended by 250 students from 40 countries.
At the London International Youth Science Forum 2010, Dr Douglas will explore future developments in science with leading scientists, dignitaries and industry pioneers. A key element of the programme is the opportunity to visit industrial sites, research centres, scientific institutions and organisations, including world-class research institutions and laboratories. For further details on how school students can participate, see: www.liysf.org.uk
References
- Shallcross D et al. (2009) Looking to the heavens: climate change experiments. Science in School 34–49: 12.
Resources
- For a comparison of the spectra of different light sources – and instructions for building your own spectrometer, see:
- Westra MT (2007) A fresh look at light: build your own spectrometer. Science in School 4: 30-34. www.scienceinschool.org/2007/issue4/spectrometer
Review
There is more to light than what we can see. The use of light is found in every aspect of science, from the brightening of the dark to the breakdown of plastic. This article gives an overview of the practical uses of light and makes interesting background reading for students researching light as a form of energy.
Andrew Galea, Malta