A classroom hydrogen economy Teach article
Could hydrogen be the best alternative for fossil fuels? This demonstration shows how a hydrogen economy might work in practice.
While fossil fuel resources are slowly being exhausted, the growing population of our planet is consuming ever more and more energy. We now know that the use of traditional carbon-containing fuels has seriously worsened environmental pollution, which makes the development of environmentally friendly energy production increasingly important.
One of the most plausible scenarios for the production of so-called ‘green’ energy is the hydrogen economy. Hydrogen has a higher energy density by weight than traditional fossil fuels and it also releases fewer greenhouse emissions. When hydrogen is burned directly or oxidised in fuel cells to obtain heat and electricity, the only product is water.
Although some companies have been developing new engines based on the internal combustion of hydrogen, fuel cells are the main energy converters on which the concept of the hydrogen economy is based.
Fuel cells were first invented in the first half of the 19th century, when British physicist William Grove suggested that if water could be split into hydrogen and oxygen by electricity, then combining the two elements could generate electricity. However, as fossil fuels became dominant, fuel cells fell by the wayside.
In the 1960s, NASA used alkaline hydrogen fuel cells in their Apollo space vehicles and later in the space shuttles to produce both electricity and water. Now the technology may get another boost.
The production and use of fuel cells are still quite limited, mainly because production and storage of hydrogen are very expensive, as are the catalysts used in the most efficient fuel cells. However, as technology improves and fossil fuels become more expensive, fuel cells are expected to replace existing energy sources and converters.
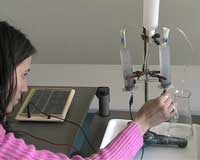
electrolyser electrodes to a
solar panel
Images courtesy of Mario Mitov
Demonstrating the principle
To explore how fuel cells work, we have developed a low-cost fuel cell for use in the classroom. The resulting electrolyser and fuel cell can be used as part of a setup to demonstrate how hydrogen might be produced and used.
The following criteria for the models were chosen:
- Materials must be accessible and cheap;
- The whole construction should be comparatively easy to allow others to observe the phenomena and processes;
- The prototypes should be safe to make.
Here we describe the materials and procedures required for constructing a water electrolyser and hydrogen fuel-cell prototype for the classroom.
Classroom activity
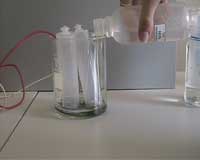
fuel cell
Images courtesy of Mario Mitov
Materials and reagents
- Plastic syringes (50 ml)
- Graphite rods or pencil leads
- Carbon gas-diffusion electrodes pressed on Ni-mesh
- Small flexible piping with valves
- T-shaped glass connector
- Plastic vessel (bottle) for the electrolyte
- Laboratory stand with clamps
- Aluminium or copper foil
- Grease-proof paper
- Hot-plate
- Glass vessels (400 ml beakers)
- Isolated copper wires with connectors
- Drill
- Silicon paste
- 6-12 V DC power source (solar panel or 9 V battery)
Figure 3: Demonstration of
the stoichiometry of the
water decomposition
Images courtesy of Mario Mitov - End consumer (low-powered electromotor with fan, LED)
- Set of resistors
- Two multimeters, or a voltmeter and an ammeter
- 1 M H2SO4
- 2 M HNO3 (for etching)
- PdCl2 solution prepared by dissolving 1 g of the salt in 50 ml of 0.5 M HCl. Heat to 50 oC and then dilute to 100 ml with distilled water.
- 1 M KOH or NaOH
- Gloves and goggles
Procedures
* The steps marked with asterisks should be performed by the teacher for safety.
Constructing the electrolyser
- *Remove the plungers and needles from two syringes.
- *Remove the rubber gaskets from the plungers and make a hole for a graphite rod electrode in each of the rubber gaskets.
- Push the graphite rods into these holes and insert the rubber gaskets back into the syringes.
- *Drill holes into the sides of both syringes about 1 cm from the bottom. The holes should be about 1 mm bigger than the outer diameter of your T-shape connector.
- Attach 1 cm lengths of hose to both edges of the T-shape connector.
- Connect the syringes with the T-shape connector.
- Use the hose to connect the electrolyte container with the free edge of the T-shape connector.
- Connect the thin flexible tubes to the narrow ends of the syringes.
- *Pour 1M H2SO4 into the electrolyte container until the electrolyte fills both syringes.
- Connect both electrodes by copper wires to the power source (figure 1).
Constructing the fuel cell
- *Remove the plungers and needles of two syringes.
- *Cut two pieces from the gas-diffusion electrodes to completely cover the flange of the syringes.
- *Place a piece of aluminium or copper foil on a hot-plate, put grease-proof paper on it, and then place the shaped gas-diffusion electrode on the paper.
- *Press the flange of a syringe onto the gas-diffusion electrode – due to the high temperature, the plastic will melt and the electrode will stick to the syringe.
- *Drill a hole through one side of the flange and the sealed gas-diffusion electrode.
- Push a metal screw through the hole.
- Remove the insulation from one end of a piece of copper wire, make several loops around the screw and tighten it with a nut.
- Isolate the bolt with silicon paste.
- Repeat steps 3 to 8 to make the second electrode.
- *Etch the electrodes by immersing them in 2 M HNO3 for 5 minutes, then wash with water.
- *Pour the solution of PdCl2 into the 400 ml beaker and immerse the prepared electrodes in it for 5 minutes. Based on the reactivity series of metals, a thin catalytic layer of elemental palladium is deposited on the gas-diffusion electrodes when palladium ions come into contact with the carbon and nickel mesh.
- *Pour 100 ml 1 M NaOH (or KOH) into another beaker and place both syringes with sealed electrodes in it (figure 2).
- Connect these syringes to the two thin hoses from the electrolyser.
After collecting some quantity of hydrogen and oxygen, stop the gas production and open the valves to allow the gases to pass to the electrodes of the fuel cell (figure 4).
Students can then measure the electrical parameters of the fuel cell by using the circuit described in figure 5. The current, I, is calculated according to Ohm’s law:
I= V/R
Instead of a resistor, a light emitting diode (LED) or low-power electromotor can also be used.
An important advantage of this system is that each of the basic modules can be replaced by other devices. For example, a special wind turbine can be used instead of a solar panel to generate the electricity that is necessary to supply the water electrolyser. Or, instead of a water electrolyser as a source of gaseous hydrogen and oxygen, gas generation by chemical reaction (figure 6) can be used.
Other versions of a fuel cell, using liquid fuel (for example, ethanol instead of hydrogen), can be also developed.
Both the electrolyser and the fuel cell in the proposed ecological energy system could be replaced by our DeMi Cell which works on the principle of reversible fuel cellsw1. Because DeMi Cells use non-dangerous salt electrolytes, they more easily satisfy safety requirements.
With some basic theoretical background, students from different educational stages are able to develop prototypes of advanced and sophisticated technologies (figure 7).
A practical demonstration

fuel cell testing
Images courtesy of Mario Mitov
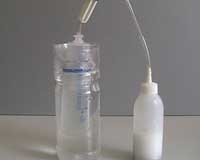
chemical gas generator and
gas holder;
Images courtesy of Mario Mitov
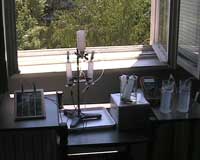
electrolyser, fuel cell and gas
holders
Images courtesy of Mario Mitov
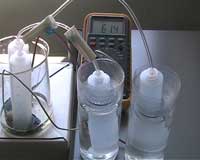
fuel cell with hydrogen and
oxygen collected in gas
holders
Images courtesy of Mario Mitov
Linking together the electrolyser and fuel cell with a solar panel, as shown in figure 1, can demonstrate how solar energy can be stored as hydrogen and then converted back into electricity. The electricity needed to power the water electrolysis can be generated by shining an artificial light source onto the solar panel, after which the evolved gases are collected above the electrolyte in the separated parts of the electrolyser (syringes). Valves stop the gases passing from the electrolyser to the fuel cells until it is needed.
The syringes also help to show that the volume of the gas from the anode is twice the volume of the gas from the cathode: 2 moles of hydrogen and 1 mole of oxygen are produced from 2 moles of water (figure 3):
(-) 4 Н2О + 4 е–→ 2 Н2 + 4 ОН–
(+) 4 ОН– → 2 Н2О + О2 + 4 е–
Summary 2 Н2О → 2 Н2 + О2
Web References
Resources
- Lex Solar provide kits with fuel cells and other renewable energy sources for school. See: www.lexsolar.de
- This book provides an introduction to the topic of fuel cells and the hydrogen economy:
- Cook B (2001) An Introduction to Fuel Cells and Hydrogen Technology. Vancouver, Canada: Heliocentris
- For more information on Mario Mitov’s work you can read
- Mitov M, Kondev I, Petrov Y, Bliznakov S, Popov A (2003) Fuel cells – Achievements and Perspectives. Khimiya 12: 455-466 (only available in Russian)
- Mitov M, Petrov Y, Manev S (2005) Demonstrational Fuel Cells. Khimiya 14: 440-445 (only available in Russian)
- Another type of fuel cell is the microbial fuel cell, which brews electricity instead of beer. You can read about how to use one of these in the classroom in
- Madden D (2010) The microbial fuel cell: electricity from yeast. Science in School 14: 32-35.
Review
This article describes a practical activity to explain the concept of the hydrogen economy, which could be used to introduce the applications of oxidation-reduction reactions. Although the activity requires materials not normally included in standard school equipment, it would be possible to obtain them quite easily.
Chemistry is sometimes seen as contributing to pollution and damage to the environment, but this text shows that it can also have a very important role in protecting the environment.
Finally, this article could also be used as a starting point to think about the advantages and disadvantages of the energy sources currently used by our society and the need to find alternatives to fossil fuel resources.
Mireia Güell Serra, Spain