What happens when cells embrace damage? Understand article
Scientists propose a new hypothesis to tackle one of the big remaining mysteries in animal evolution.
Kintsugi is an ancient Japanese art of repairing broken ceramics with gold or other precious materials. The underlying philosophy is that breakage and repair are integral parts of an object’s life, and they can actually result in more valuable items. According to a hypothesis developed by scientists Detlev Arendt and Thibaut Brunet at the European Molecular Biology Laboratory (EMBL)w1 in Heidelberg, Germany, ancestral eukaryotic cells might have undergone a similar process of damage and repair that, over the course of evolution, gave rise to highly specialised brain and muscle cells (Brunet & Arendt, 2016).
A cellular poison
When a neuron or a muscle cell in our body detects a signal, it responds by allowing some ions, such as sodium and calcium, to enter through the cell membrane. These inward flows of molecules trigger precise responses, which cause muscle cells to contract and neurons to release chemicals responsible for cell-to-cell communication. How our central nervous system evolved to use these chemical triggers is still a mystery, but Detlev and Thibaut are edging closer to an answer.
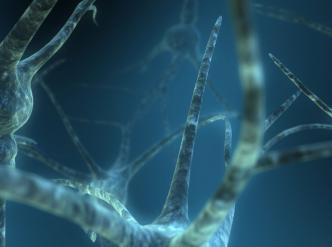
known as dendrites, transmit impulses to the cell body.
Image courtesy of Scott Ingram; image source: Flickr
At high concentrations, calcium is harmful for all living cells, as it forms insoluble aggregates with molecules that are key for cell survival. This is why calcium can be 10 000 times less abundant inside the cell than it is in blood. The chemical composition of modern cells is thought to be a ‘fingerprint’ of the composition of the environment in which life began more than 3.5 billion years ago. As they were permeable to small molecules, the first cells had the same chemical composition as the aquatic environment in which they lived, where the concentration of calcium was low. But when ancestral cells first became exposed to environments rich in calcium, probably around 1.5 billion years ago, they had to develop a response to protect themselves from this toxic molecule. Since one of the major routes of calcium influx is cell membrane rupture, the scientists believe that a very important cellular response had to be counteracting membrane damage.
“If you look at eukaryotic cells, from algae to neurons, the majority of the molecules that make a cell contract are sensitive to calcium, so we speculated that cell constriction first evolved in response to an influx of external poison to close the membrane upon breakage,” says Thibaut. “This was a bit of a shot in the dark, but when we looked at the literature to test this hypothesis, we were excited to see it is actually the way it works!”
The path to neurons and muscles
Following a rupture of the cell membrane, two calcium-sensitive proteins, called actin and myosin, organise in a ring around the membrane wound. The mesh created by actin and myosin shrinks, causing the cell membrane to constrict and gradually seal the wound. Calcium also triggers the release of bubble-like structures called vesicles. These vesicles are rich in fatty molecules, which provide the broken cell membrane with new building blocks. The scientists believe that this wound-healing mechanism could have been the ancestral cells’ first ‘emergency response’ to calcium influx.
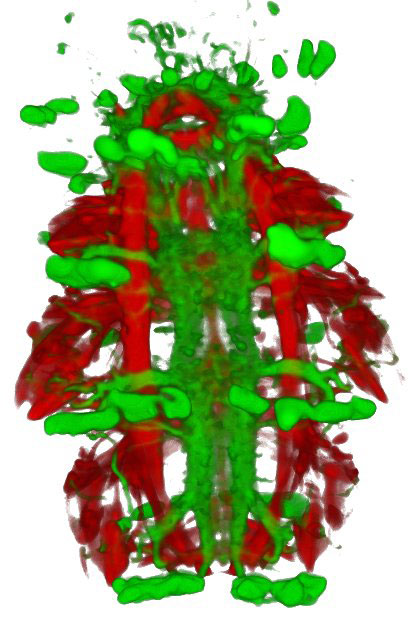
Platynereis: muscles are
shown in red and the
nervous system in green.
Image courtesy of Thibaut
Brunet / EMBL
As is often the case in evolution, this mechanism – which had evolved originally for membrane repair – turned out to be useful in other contexts. Membrane contraction, which deforms the cell, became a way for cells to move. The release of molecules by secretion from vesicles became a powerful way to communicate with other cells.
As they increased in complexity, ancestral cells built systems that control contraction and secretion by mimicking membrane rupture, promoting a controlled calcium influx. This system developed further, and ion influx could be actively amplified into an action potential – a short electrical signal that can rapidly spread across the cell membrane, triggering the activation of contraction and secretion. Ancestral cells were now multitasking: they were able to generate an action potential that caused a portion of the same cell to contract. Action potentials also stimulated the release of vesicles packed with chemicals necessary for cell-to-cell communication, which resulted in the spreading of the contractile activity to neighbouring cells.
Some of these cell progenitors later gave rise to two distinct cell types through the division of labour: they literally split into two branches, contractile cells and mechanosensory cells. On one branch, contractile cells, whose primary activity was contracting, have evolved into muscle cells. On the other branch, mechanosensory cells, which specialised in generating action potentials and releasing cell-to-cell communication vesicles, have evolved into the neurons we see today. But the ancient pathways were maintained: even now, our neurons and muscles still rely on a controlled influx of a strong cellular poison – calcium – to decide when to secrete and to contract.
References
- Brunet T, Arendt D (2016) From damage response to action potentials: early evolution of neural and contractile modules in stem eukaryotes. Philosophical Transactions of the Royal Society of London Series B, Biological Sciences 371: 20150043
Web References
- w1 – Read more about the Arendt Group at EMBL.
Institutions
Review
Can you think about a difficulty you managed to overcome that ultimately made you stronger? According to this article, ancient eukaryotic cells may have undergone damage and repair processes that led to more specialised cells – thanks to evolution.
The ideas mentioned in the article could be used in biology lessons in secondary school, particularly when studying biochemistry and evolution. The text also highlights the fact that chemical reactions and physical processes have an essential role in cellular processes.
Before reading the article, students could think about what triggered the evolution of simple eukaryotic cells to more complex ones.
Apart from being used as a comprehension exercise, this article can help to raise awareness of the importance of evolution in the development of life on our planet. Potential discussion questions include:
- How do neurons or muscle cells in the human body react when they detect a signal?
- Why are high concentrations of calcium harmful for all living cells?
- What is the role of actin and myosin proteins following cell membrane rupture?
- How do cells communicate with one another?
- What is an action potential?
Mireia Güell Serra, Spain