Click does the trick: understanding the 2022 Nobel Prize in Chemistry Inspire article
Why was a Nobel prize awarded for 'click chemistry'? Learn about the ground-breaking advance behind this simple-sounding name.
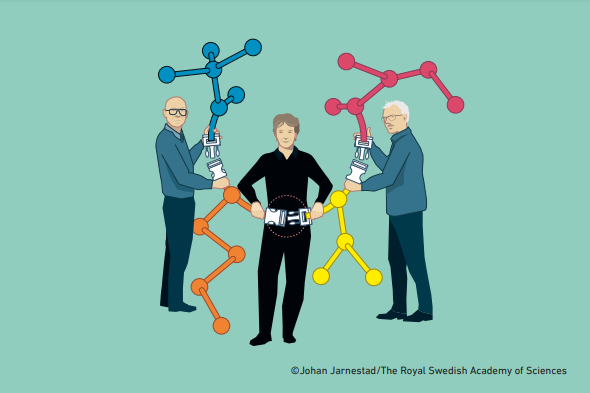
This year’s Nobel Prize in Chemistry was awarded to Carolyn R. Bertozzi, Morten Meldal, and K. Barry Sharpless for the development of click chemistry and bioorthogonal chemistry.[1] This came as no surprise to chemists and biologists; in fact a Nobel prize for this work had been expected for some time. Often, Nobel prizes are awarded for rather specialized research that is very important but relatively unknown outside of that particular research topic. Click chemistry, however, has revolutionized chemical and biological research to such an extent that most scientists in these fields are aware of it and many will have used it in their research. I’ve used this chemistry myself in the lab, and I’ve read many inspiring research papers that have relied on it as a fundamental technique. But what are click chemistry and bioorthogonal chemistry, and why have these techniques turned out to be such an important advance?
What is click chemistry?
When talking recently to a non-scientist colleague about this year’s Nobel prize, she commented that click chemistry sounds childishly simple, “like Lego.” She actually had the fundamental advance of this work spot on! Click chemistry is so exciting and has proven so useful exactly because it is simple and modular. Barry Sharpless defined click chemistry as reactions that are “modular, wide in scope, give very high yields, and generate only inoffensive byproducts”.[2] But what does this mean, and why are these properties important?
Characteristics of click reactions
Modularity – small, simple reactive groups that can easily be added to larger molecules to join different kinds of molecules together
Wide scope – compatibility with a wide range of functional groups, i.e. other parts of the molecule will not hinder the reaction or be harmed by it
Simple, mild reaction conditions – ideally room temperature and atmospheric pressure, and without the need for several steps or purifications
High yields – the reactions should also ideally proceed rapidly to completion. A high thermodynamic driving force is often used to achieve this
Specificity – reactions should take place only at the intended functional group and give predictable products
No harsh solvent – ideally, they should take place in aqueous solvent (water) rather than organic solvents, or at least be compatible with water and oxygen
Inoffensive products – there should either be no byproducts, or these should be nontoxic and unreactive or easy to remove
Stable products – they should not react further or break down under physiological conditions, and they should be easy to isolate
The best-known click reaction is copper(I)-catalyzed azide–alkyne cycloaddition (CuAAC), and was discovered independently by the groups of Barry Sharpless[3] and Morten Meldal.[4] This reaction does exactly what it says on the tin: an azide and an alkyne react directly in the presence of copper to give a stable cyclic triazole reaction product.
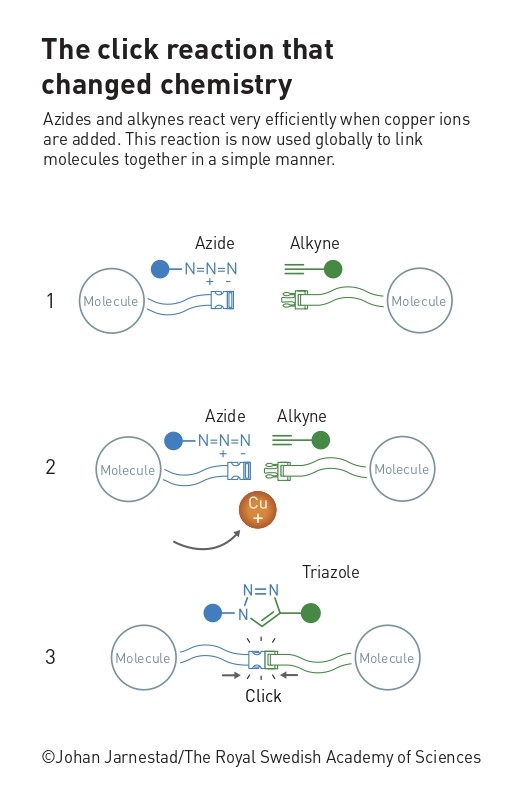
Why is click chemistry so useful?
In some ways, the most interesting parts of this reaction are the molecules to which the click reactive groups, or ‘handles,’ are attached. These can be almost anything, from proteins to drug fragments to nanoparticles. Many chemical reactions used to connect molecules require harsh (and environmentally unfriendly) reaction conditions, such as high pressure or temperature, harmful organic solvents that must be carefully removed afterwards, or multiple steps with purifications in between. Furthermore, they are often unselective (give a mix of products), incompatible with certain functional groups elsewhere in the molecules, don’t function if water or oxygen are present, give low yields, or produce toxic or reactive byproducts that are difficult to separate. The disadvantages of these features are obvious, and click reactions have allowed scientists in wide-ranging fields to synthesize and modify compounds more easily. For example, they can be used to create compound libraries for drug development,[5,6] or to build or functionalize polymers and nanomaterials.[7] Importantly, the simple, modular nature of click reactions means that even people who are not experts in organic chemistry can order the building blocks and use these reactions in their research.
The mild aqueous reaction conditions, specificity, and broad scope of click chemistry have proven stunningly useful in bioconjugation, that is, reactions with biomolecules like proteins, carbohydrates, lipids, and nucleic acids. These complex molecules have many functional groups, are difficult to modify specifically, and are often destroyed by organic solvents or harsh reaction conditions. Why would we want to chemically modify biomolecules? There are many reasons, and a particularly important one is labelling.[8,9] Our article on fluorescence microscopy gives an example of this. It is important to be able to trace where specific biomolecules are located in cells or tissues to understand their function in health and disease. Bioconjugation reactions enable the attachment of fluorophores, which can then be tracked with fluorescence microscopy. Many other types of tag or label can also be attached, for example, for purification or medical imaging. Another use of bioconjugation is targeting, where a drug compound is attached to a targeting group to ensure that it reaches the correct protein, cell, or tissue (e.g., a tumour). These targeting approaches could reduce toxic side effects by reducing the amount of compound needed and ensuring that it doesn’t act in the wrong place.
What about bioorthogonal chemistry?
While CuAAC is compatible with biomolecules, it can’t be used in living systems because copper is toxic. This is where the second part of the Nobel prize comes in. Carolyn Bertozzi coined the term ‘bioorthogonal’ for reactions that can be carried out in biological systems without interfering with, or being affected by, any surrounding biological processes. She was awarded the prize for developing reactions that don’t require a toxic metal catalyst and can be used in living cells, in particular, strain-promoted azide–alkyne cycloaddition (SPAAC).[10] In this case, the ring strain in the difluorooctyne starting material destabilizes it to such an extent that the reaction proceeds efficiently with no catalyst.
This has opened up new avenues for the use of click chemistry to investigate cell biology. Techniques like genetic code expansion or metabolic engineering can be used to induce cells to make biomolecules with a click handle attached.[11] These biomolecules can then be modified in the living cells by adding a click-modified reaction partner, which allows scientist to influence and track biological processes in real time. The Bertozzi group has used these reactions to investigate glycosylation (the addition of sugars or carbohydrates to biomolecules in the cell), which is an extremely important cellular process with many implications for health and disease.[12]
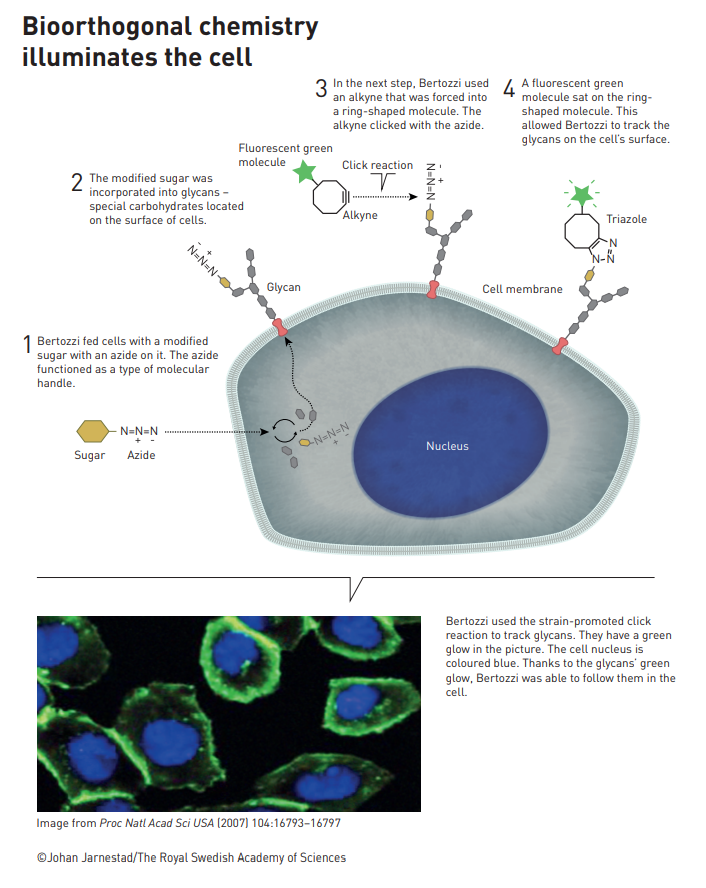
Summary
It is difficult to overstate the impact that click chemistry and bioorthogonal chemistry have had on science, particularly in physiological, biochemical, biomedical, and materials research. A number of additional click and bioorthogonal reactions have been developed since this ground-breaking work, and these chemical tools have enabled countless important discoveries and advances. This really highlights the multidisciplinary nature of modern research. The initial work was done by organic chemists and is now widely used across many different fields, from cell biology to drug development to polymer science. There is a great deal of public misconception regarding what kinds of research are important. People often feel that research that does not directly address a societal need, such as the development of medicines or technology, is a waste of money. However, method development and basic research to gain fundamental knowledge are crucial building blocks for the life-changing applications of the future.
References
[1] Carolyn R. Bertozzi, Morten Meldal, and K. Barry Sharpless are awarded the Nobel Prize in Chemistry, 2022: https://www.nobelprize.org/uploads/2022/10/advanced-chemistryprize2022-2.pdf
[2] Kolb HC et al. (2001) Click Chemistry: Diverse chemical function from a few good reactions. Angewandte Chemie 40: 2004–2021. doi: 10.1002/1521-3773(20010601)40:11<2004::AID-ANIE2004>3.0.CO;2-5
[3] Rostovtsev VV et al. (2002) A Stepwise Huisgen Cycloaddition Process: Copper(I)-Catalyzed Regioselective “Ligation” of Azides and Terminal Alkynes. Angewandte Chemie 41: 2569–2599. doi: 10.1002/1521-3773(20020715)41:14<2596::AID-ANIE2596>3.0.CO;2-4
[4] Tornøe CW, Christensen C, Meldal M (2002) Peptidotriazoles on Solid Phase: [1,2,3]-Triazoles by Regiospecific Copper(I)-Catalyzed 1,3-Dipolar Cycloadditions of Terminal Alkynes to Azides. The Journal of Organic Chemistry 67: 3057–3064. doi: 10.1021/jo011148j
[5] Hein CD, Xiu X, Wang D (2008) Click Chemistry, a Powerful Tool for Pharmaceutical Sciences. Pharmaceutical Research 25: 2216–2230. doi: 10.1007/s11095-008-9616-1
[6] Sharpless KB, Manetsch R (2006) In situ click chemistry: a powerful means for lead discovery. Expert Opinion on Drug Discovery 1: 525–538. doi: 10.1517/17460441.1.6.525
[7] Geng Y et al. (2021) Click chemistry strategies for the accelerated synthesis of functional macromolecules. Journal of Polymer Science 59: 963–1042. doi: 10.1002/pol.20210126
[8] Kaur J, Saxena M, Rishi N (2021) An Overview of Recent Advances in Biomedical Applications of Click Chemistry. Bioconjugate Chemistry 32: 1455–1471. doi: 10.1021/acs.bioconjchem.1c00247
[9] Zuin Fantoni N, El-Sagheer AH, Brown T (2021) A Hitchhiker’s Guide to Click-Chemistry with Nucleic Acids. Chemical Reviews 121: 7122–7154. doi: 10.1021/acs.chemrev.0c00928
[10] Agard NJ et al. (2006) A Comparative Study of Bioorthogonal Reactions with Azides. ACS Chemical Biology 1: 644–648. doi: 10.1021/cb6003228
[11] Nikić I, Lemke EA (2015) Genetic code expansion enabled site-specific dual-color protein labeling: superresolution microscopy and beyond. Current Opinion in Chemical Biology 28: 164–173. doi: 10.1016/j.cbpa.2015.07.021
[12] Agard NJ, Bertozzi CR (2009) Chemical Approaches To Perturb, Profile, and Perceive Glycans. Accounts of Chemical Research 42: 788–797. doi: 10.1021/ar800267j
Resources
- Read the Nobel Prize Organization’s explanation of the importance of click chemistry and bioorthogonal chemistry.
- Read an article from The guardian about the 2022 Nobel Prize in Chemistry.
- Try this role-playing activity to understand how research projects are funded and the importance of basic research: McHugh M et al. (2021) What is it good for? Basic versus applied research. Science in School 55.
- Take the mystery box challenge to learn about the nature of science: Horvat AK et al. (2022) The mystery box challenge: explore the nature of science. Science in School 59.
- Learn how fluorescence microscopy can illuminate our gut microbiome: Ponnudurai RP (2022) Shedding light on the gut microbiome. Science in School 60.
- Read an interview with the winner of this year’s Nobel Prize in Physiology or Medicine, Svante Pääbo: Hayes E (2011) An archaeologist of the genome: Svante Pääbo. Science in School 20: 6–12.
- Read an interview with the winner of the 2001 Nobel Prize in Physiology or Medicine, Tim Hunt: Gebhardt P (2007) Eyes on the horizon, feet on the ground: interview with Tim Hunt. Science in School 18: 9–13.