Cells: why shape matters Understand article
New techniques are revealing how cells control their shape – and the changes that could give an early warning of disease.
Billions of years of evolution have given living organisms an amazing diversity of shapes and forms, from the amorphous irregularity of simple animals such as sponges, to the sculpted and symmetrical shapes of more complex creatures, including ourselves. But it is not just organisms’ bodies that have a great range of shapes: their cells do, too.
The shapes of cells usually relate to their function. The neurons in our brains, for instance, have star-like branches to network with other neurons, and red blood cells have the form of biconcave discs to maximise their oxygen-carrying capacity while allowing them to squeeze through the narrowest blood vessels. In contrast, macrophages (a type of white blood cell) have changing, amoeba-like shapes that help them engulf foreign bodies. In the plant world, cell shapes vary from the elaborate spiny forms of pollen grains – adapted to catch the wind or adhere to pollinating insects – to the expandable kidney shape of guard cells, which open and close pores in leaves. Cells can also take more complicated shapes, like twisted prisms or ‘scutoids’; these are nature’s way of allowing epithelial tissues to curve.
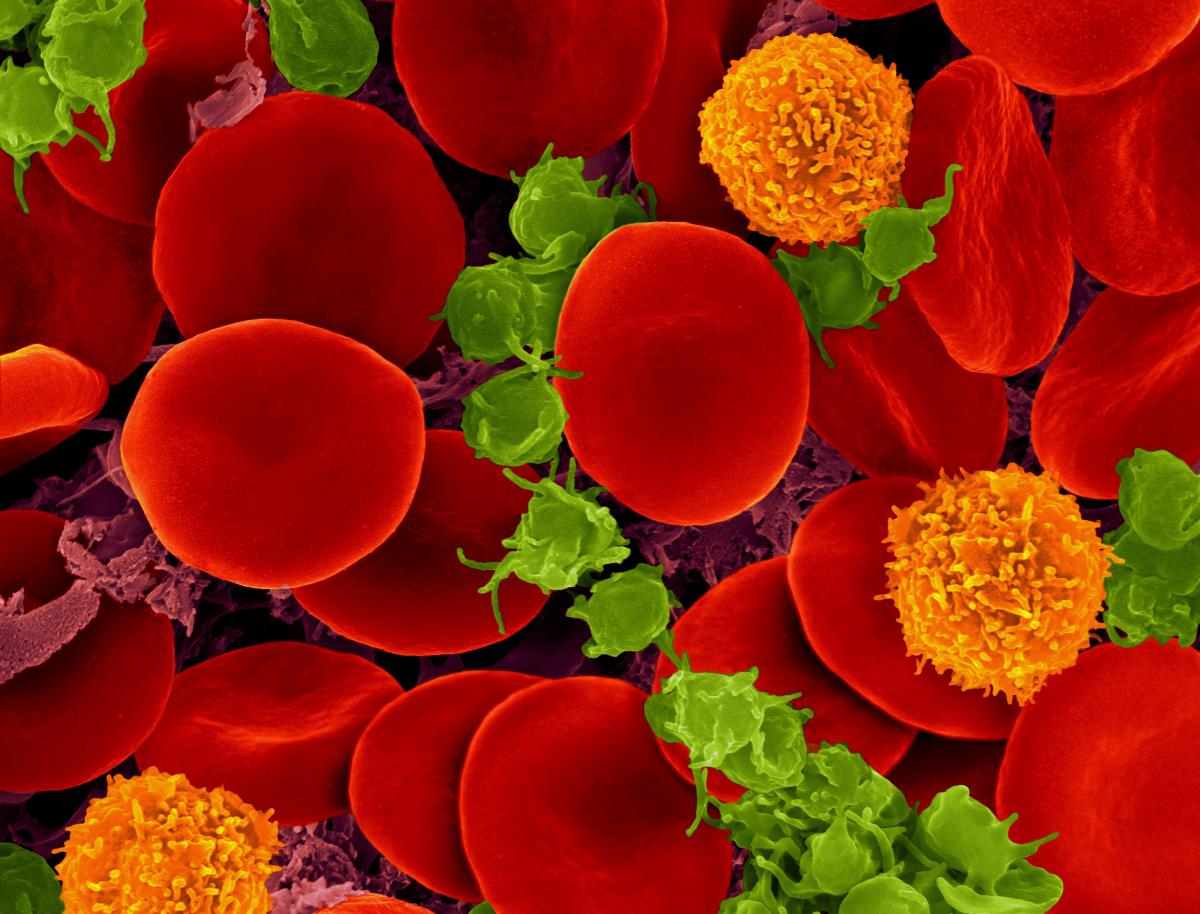
ZEISS Microscopy/Flickr, CC BY-NC-ND 2.0
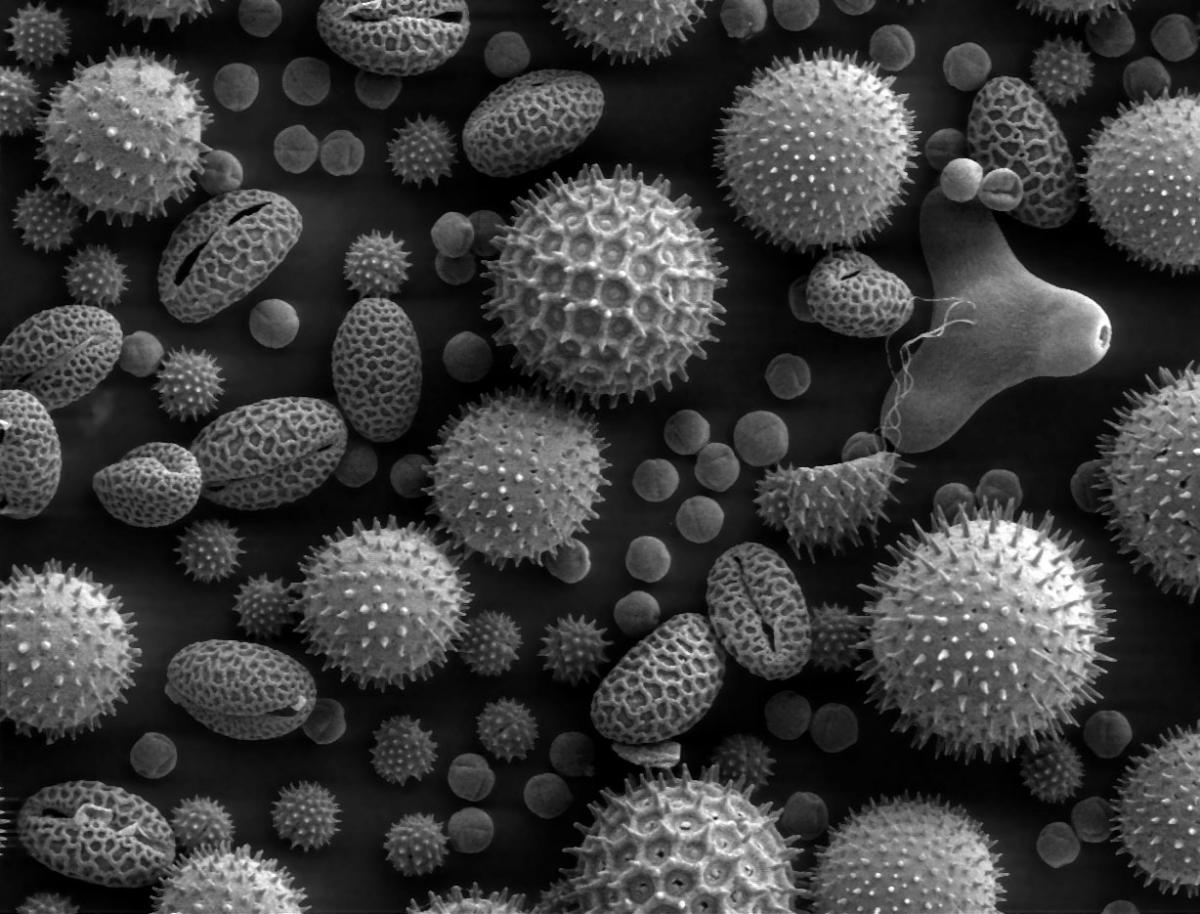
Dartmouth College Electron Microscope Facility/Wikimedia Commons, CC0
Changing shapes
Although a cell’s shape is linked to its functional role, many cells have the potential to change their shape. This ability is critical in embryonic development, when tissues undergo dramatic folding, furrowing and bending transformations as organs are generated. In fruit fly embryos, for example, the process of gastrulation – during which an embryonic ball of cells begins to differentiate into distinct tissues – is driven by a change in cell shape known as apical constriction. Epithelial cells on one side of the embryo change from column-shaped to bottle-shaped, causing the epithelium to wrap itself into a tube (figure 1).
Major changes in cell shape also occur when clusters of cells migrate from one part of an embryo to another. In zebrafish embryos, cells involved in the development of the ‘lateral line’ (a sensory organ found in fish) grow tiny mobile protrusions called filopodia and lamellipodia. They appear on the leading edge of the cluster and seem to lead the entire cluster in a particular direction (figure 2).
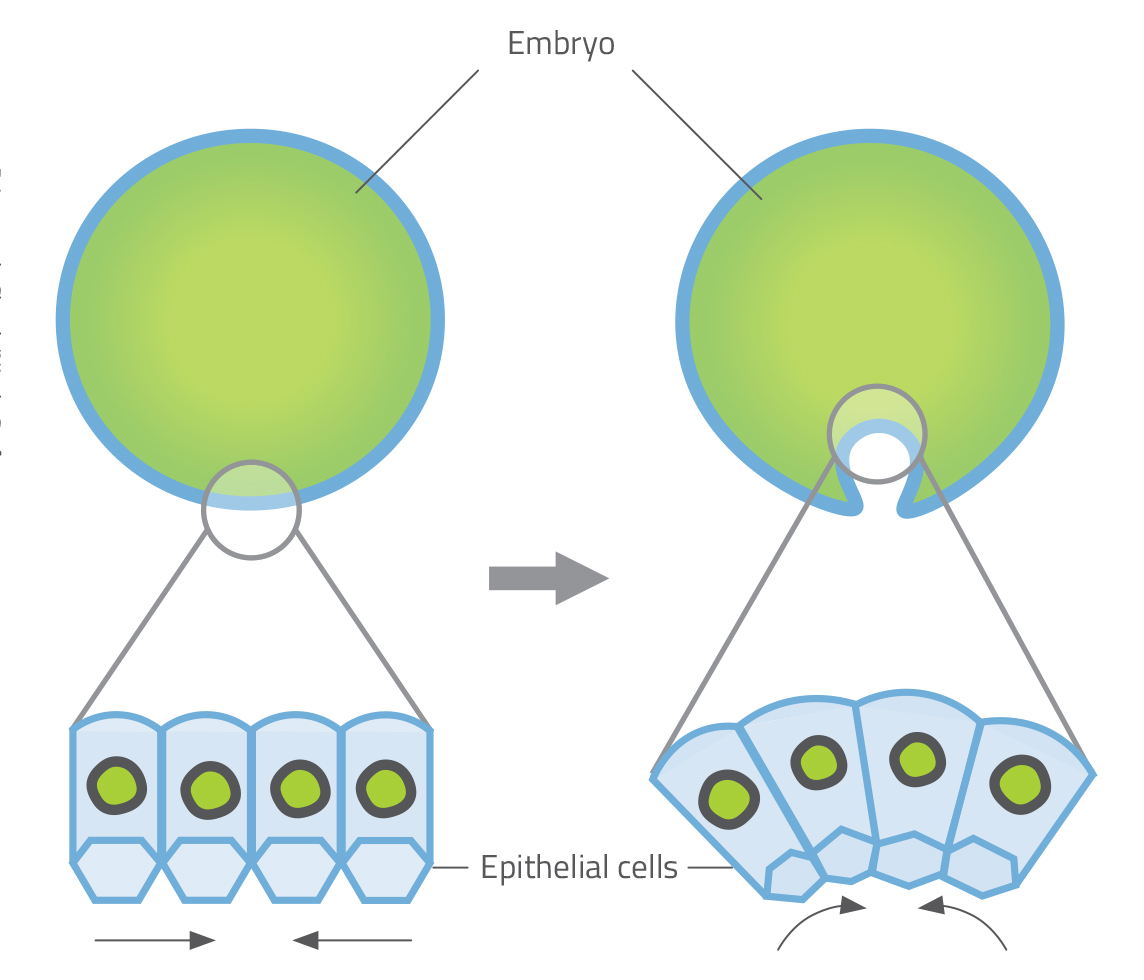
Priyamvada Chugh/Nicola Graf
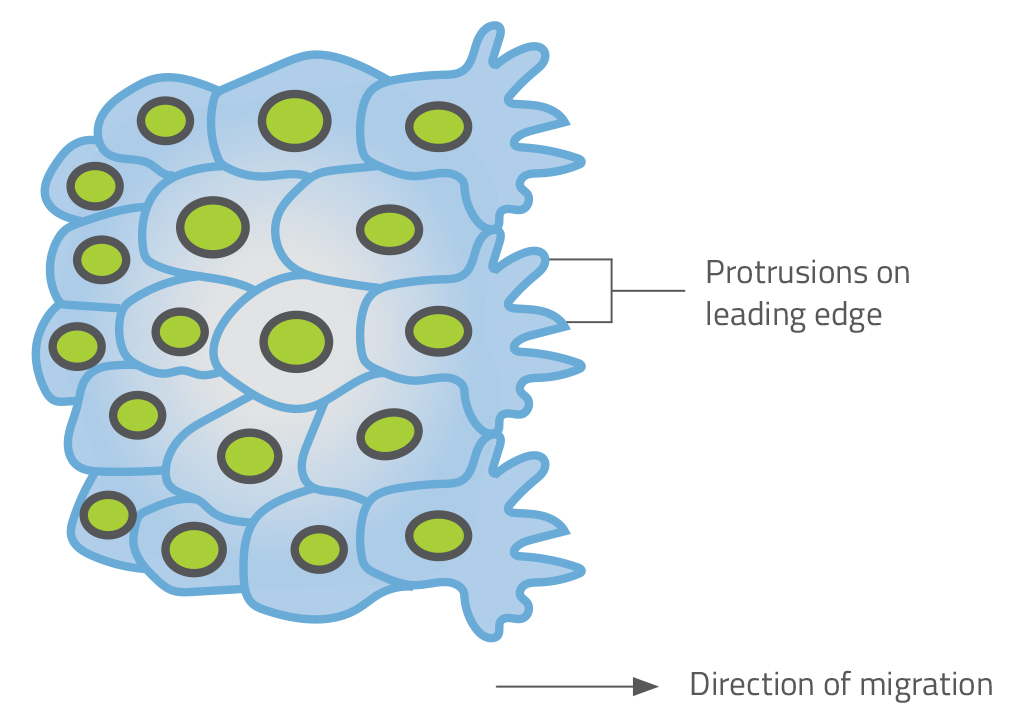
Priyamvada Chugh/Nicola Graf
Shape change is also important in cell division, which plays a crucial role in many developmental processes. When mitosis (cell division) begins, cells change from flat to spherical. Experiments that confined dividing cells inside microscopic channels, restricting their dimensions, revealed that the spherical stage is essential for chromosomes to be distributed equally among daughter cells. If this shape change is prevented, the cell division fails (Lancaster et al., 2013).
Controlling cell shape
But what exactly is it that controls cell shape? Although we don’t yet have a comprehensive understanding, we have discovered some of the molecular and mechanical processes that determine a cell’s shape. These findings come largely from familiar laboratory organisms such as fruit flies and zebrafish, or from cancer cells grown in culture, but many of the processes involved are likely to be universal.
One important part of a cell that influences its shape is the cortex – a network of structural proteins that forms a layer beneath the cell’s outer membrane. The physical properties of the cortex determine how rigid or soft the cell’s surface is and thus how malleable the cell is as a whole. The cortex consists mainly of two kinds of protein that are closely related to the proteins that make muscle fibres contract: actin, which forms long microfilaments; and myosin, which binds to the actin microfilaments and uses chemical energy to pull them, acting like a motor. This system generates contraction in a similar way to the actomyosin system in muscles.
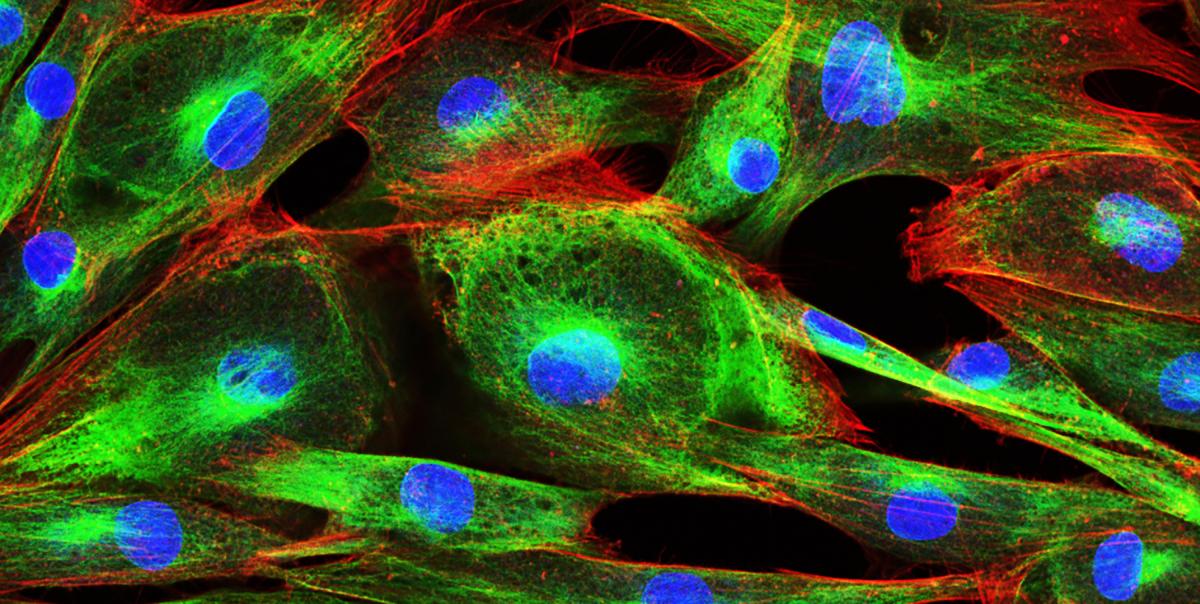
Vshivkova/Shutterstock.com
Scientists have also identified hundreds of regulatory proteins that interact with the actin microfilaments in the cortex. Finding out how these proteins affect the cortex, and thus the cell’s shape, is an active area of research. One common technique used to study them is to block the production of regulatory proteins one at a time using siRNAs (small interfering RNA molecules), which silence particular genes. Other experiments have investigated how switching off the myosin ‘motors’ or chopping actin microfilaments into fragments affects cell shape.
A key finding is that the actomyosin cortex controls cell shape by creating tension in the cell surface, rather like the tension in the skin of a balloon. If the myosin motors are inhibited or the actin filaments are broken down, the tension is lost and the cell surface forms bulges, disrupting its shape.
In many cases, the shapes of cells are dictated by external forces. For instance, epithelial cells within the wing of a fruit fly form hexagonal shapes because they are packed together in a tight honeycomb, with each cell surrounded by six or so neighbours. Similarly, the cells that line our blood capillaries are sculpted by the flow of blood, causing them to become elongated and lined up parallel to the flow.
Cell shape and disease
Changes in cell shape have a key role in the development of many diseases, including those caused by infectious organisms and others triggered by faulty genes. For example, cancer-causing mutations can make cells lose the adhesions that bind them to neighbouring cells, making them amorphous and deformable – and thereby helping them to travel to and grow in other parts of the body. In Alzheimer’s disease, mutations affect the twig-like dendrites that wire brain cells together, making them shorter or less finely branched. In sickle cell anaemia, a mutation gives red blood cells a curved, sickle shape, while in malaria – a disease caused when the Plasmodium parasite infects red blood cells – the cells become stiffer and less deformable.
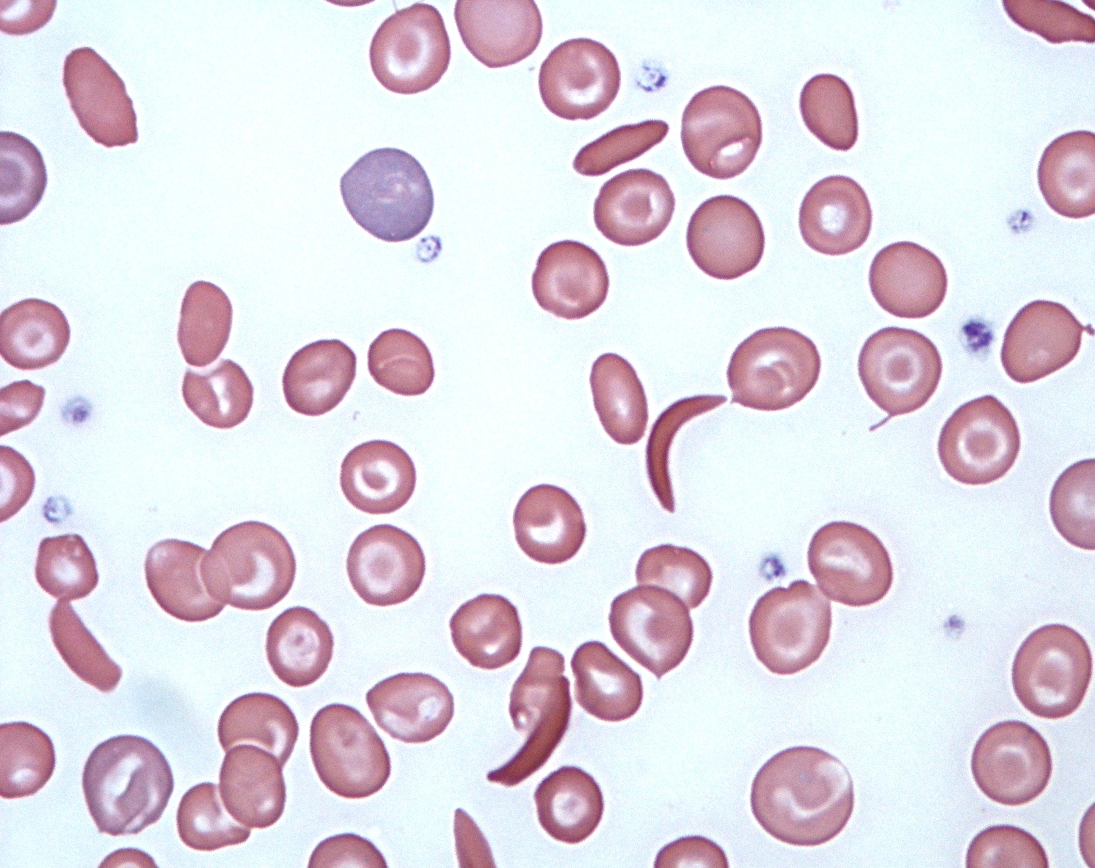
Viv Caruna/Flickr, CC BY-2.0
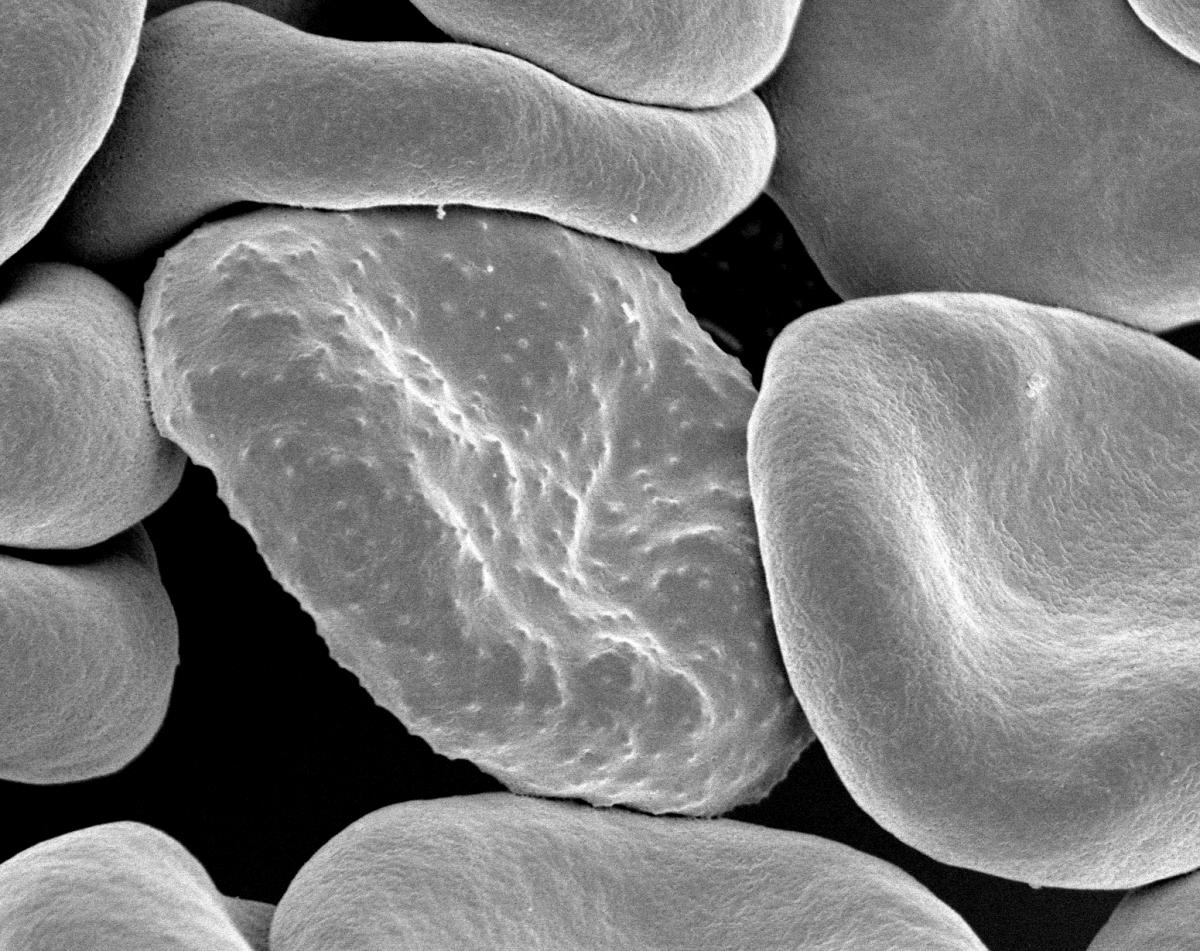
Rick Fairhurst/Jordan Zuspann/National Institute of Allergy and Infectious Diseases/National Institutes of Health/Flickr, CC BY-NC 2.0
Although we now know that abnormalities in cell shape are seen in some of the most common and hard-to-treat diseases (such as cancer and Alzheimer’s disease), it is not always clear whether these defects have a causal role or are merely symptoms of disease. However, the study of cell shape, and new techniques that support this, offer a new line of enquiry for investigating how such diseases develop.
For example, collaboration between biologists and physicists has yielded a technique called real-time deformability cytometry, or RTDC (see text box). As well as advancing our understanding of cell shape and its role in development and disease, this new technique may prove to be an effective way of detecting cancer cells at an early stage. Such techniques offer the hope of new diagnostic methods that could save lives by detecting disease earlier, when treatment has a much better chance of success.
Cell shape technology
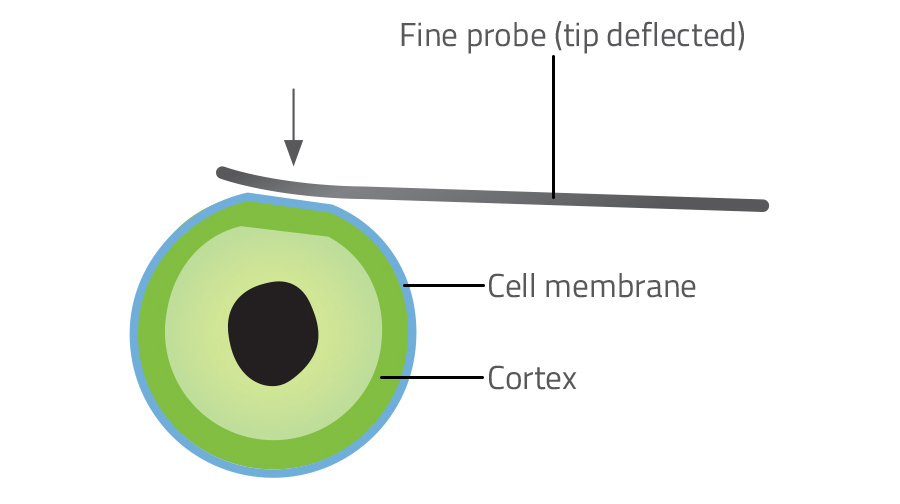
Priyamvada Chugh/Nicola Graf
New technologies have made it possible to measure the tension in the surface of individual cells, which in turn tells us how much tension there is in the underlying cortex. The three most common techniques for measuring cell surface tension are atomic force microscopy, micropipette aspiration and laser ablation.
Atomic force microscopy uses a tiny metal tip, finer than a hair, to probe the cell surface (figure 3). The amount of deflection in the probe reveals how stiff the surface is. Using this technique, scientists discovered that an increase in tension drives the change in shape from flat to spherical in the early stage of cell division. Micropipette aspiration involves measuring the force required to suck a portion of a cell into a microscopic pipette (figure 4). Laser ablation involves severing the network of actin microfilaments in the cortex with a laser and measuring the subsequent recoil as the two sides of the cortex snap back like cut rubber bands.
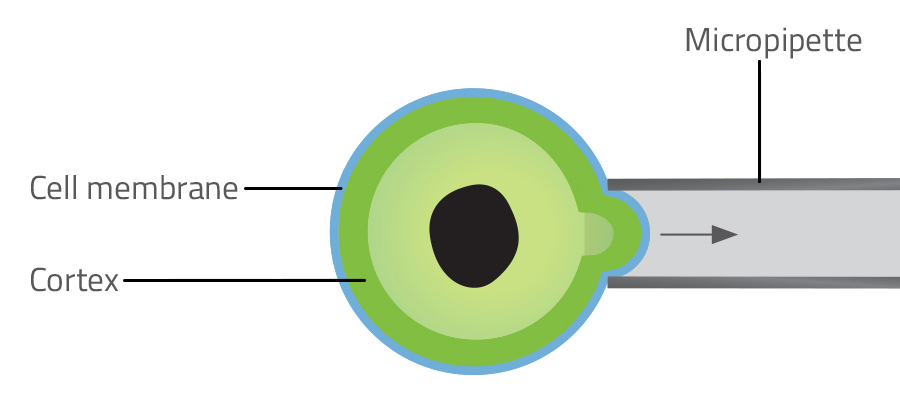
Priyamvada Chugh/Nicola Graf
Although these three methods are all effective, they assess only one cell at a time. Another technique, real-time deformability cytometry (RTDC), can assess hundreds of cells per second (Otto et al., 2015). In RTDC, a suspension of cells is pumped by syringe through a microscopic channel. Forces in the flowing liquid cause abnormally soft cells – such as cancer cells – to deform, while more rigid cells are less affected. A camera hooked up to a microscope records images of the passing cells, and these are processed by software.
Advances in optics have also made it possible to capture detailed 3D images of living cells and even see individual protein molecules inside them, using laser-scanning microscopes. These super-resolution microscopes have revealed that the actin filaments of the cortex organise themselves into parallel bundles at the cell equator during mitosis, maximising the tension they generate and so pinching the cell into two daughter cells.
References
- Lancaster OM et al. (2013) Mitotic rounding alters cell geometry to ensure efficient bipolar spindle formation. Developmental Cell 25: 270–283. doi: 10.1016/j.devcel.2013.03.014
- Otto O et al. (2015) Real-time deformability cytometry: on-the-fly cell mechanical phenotyping. Nature Methods 12: 199–202. doi: 10.1038/nmeth.3281
Resources
- Read about the newly discovered ‘scutoid’ cell shape on the New Scientist website.
- Watch a video of a neutrophil (a type of white blood cell) changing shape as it chases bacteria. Visit the Embryology education and research website.
- Read an accessible overview of the many shapes and sizes of neurons, the signalling cells of the nervous system, from the Scientific American blog.
Review
The link between cell shape and function is fundamental to understanding cell and tissue biology. This article offers a glimpse into the world of cell biology, showing how important cell shape is in the development of organisms, tissue shape and function, and as a disease marker.
The article would be useful as wider reading in cell biology for older students. This could be linked to observing cells and tissues under the light microscope. The introduction of more molecular biology into higher-level school curricula gives an opportunity to introduce the functions of siRNA molecules, which are mentioned in the article. Finally, students could use the idea of cell shape as a disease marker to develop a poster of cell types and shapes, showing how cell shape changes with disease and how this is used in diagnosis.
Dr Shelley Goodman, college lecturer in applied science, UK