On your bike: how muscles respond to exercise Understand article
We all know that exercise makes us fitter and healthier – but what changes take place in our cells to make this happen?
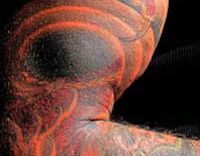
Compete; image source: Flickr
Next time you are working out in the gym, or pounding the streets running or jogging, ponder this: the idea of ‘muscle memory’ – that today’s exercise has effects on our muscles years from now – has never been demonstrated scientifically. Does it really exist, and if so, how does it work?
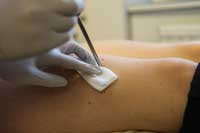
used to take a fragment of
muscle from the leg of a
study participant. This tissue
can reveal what changes
occur in muscle fibres in
response to different types
of exercise
Image courtesy of Maléne
Lindholm and Susanna Wallman
Appel
These are some of the questions we hope to answer in our on-going research, which aims to pin down the changes that occur in muscles when we exercise, and how our muscles ‘know’ to respond differently to, say, endurance training as opposed to strength training.
Helping us to investigate these questions is a large team of volunteers. Not only must they cycle to exhaustion in our gym, but before and after a strenuous exercise regime lasting several weeks, we take a tiny sample of their leg muscle under local anaesthetic (figure 1). The aim of our research is to help people optimise their training programmes for maximal fitness, and potentially to help develop new treatments for people who cannot exercise because they are paralysed or have joint diseases.
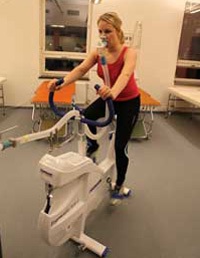
studies, participants perform
a test in which all their
exhaled air is collected and
the amount of oxygen taken
up by the body is measured.
This provides information on
the fitness level of each
participant’s heart and
working muscles
Image courtesy of Maléne
Lindholm and Susanna Wallman
Appel
We assess the fitness of our volunteers before and after participation in the studies by measuring their maximal oxygen uptake. They cycle on an exercise bike against increasing resistance until exhausted, while wearing a mask to analyse their oxygen consumption (figure 2). This gives us information about the pumping capacity of the heart and metabolism of the working muscles – both factors associated with a person’s fitness level.
Then we study the muscle tissue from the biopsies either by slicing and staining the tissue, then viewing it under a microscope (figure 3), or by breaking up the tissue and measuring the levels of particular molecules.
Of course, we already know that regular exercise produces health benefits. Physically active individuals have a lower risk of developing cardiovascular diseases, type II diabetes and certain types of cancer. Even a moderate amount of daily physical activity, for example 30 minutes of brisk walking, is enough to confer many of the benefits. And the more exercise we take, the greater the benefits.
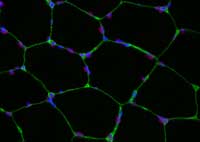
used to visualise changes
that occur within the muscle
fibres in response to
exercise. Different types of
staining can reveal different
structures within the cell.
Here, one specific histone
modification is stained in red,
with the nuclei in blue and
the cell membrane in green.
To find out if a change has
occurred, we would compare
it with an image taken before
training. Click on image to
enlarge
Image courtesy of Maléne
Lindholm and Susanna Wallman
Appel
It’s not just a question of how much exercise we take, though, but also what sort and how intense it is: different types of exercise produce different effects on the body. Heavy resistance training, such as weight lifting, causes skeletal muscles to grow, providing enhanced strength, whereas regular endurance exercise, for example long-distance running, cycling or aerobics, improves fitness and reduces fatigue.
How does regular endurance exercise lead to these effects? Over time, the heart gains the ability to pump larger strokes and after a couple of months of training, new tiny blood vessels (capillaries) form around muscle cells to ensure a good oxygen supply. Also, the number of mitochondria – the cell’s ‘power plants’ – increases. Inside the mitochondria, enzymes use oxygen to turn digested sugar and fat into usable energy. The more mitochondria the muscles have, the more fat and sugar they can metabolise and the more energy they can release.
But what we don’t yet understand is exactly how exercise causes such changes. We are pursuing this question along two lines: first, how does exercise lead to more mitochondria in skeletal muscle cells? And second, how does exercise change the way in which the cell’s DNA is used?
Building mitochondria
Mitochondria are constructed from protein molecules, so factors that boost the production of mitochondrial proteins can increase the number of mitochondria in a cell. One factor that acts as a key regulator of the production of mitochondrial proteins is a molecule called PGC-1α (figure 4).
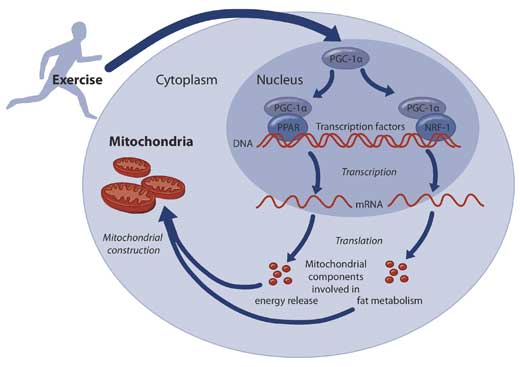
Click on image to enlarge
Image courtesy of Susanne Mükusch
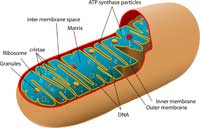
cross- section of a
mitochondrion, showing its
inner folded membrane
structure. Click on image to
enlarge
Image courtesy of Mariana Ruiz
Villarreal; image source:
Wikimedia Commons
For a gene to be expressed – that is, used to make a protein – the DNA information held in the nucleus must first be copied, or transcribed, onto an mRNA molecule. The mRNA molecules then move out of the nucleus to sites in the cell where protein molecules are constructed.
The transcription process is controlled by DNA-binding molecules called transcription factors. These attach to the DNA strand at very specific points, either blocking or promoting the transcription process. PGC-1α acts in concert with transcription factors to promote the expression of many genes coding for mitochondrial proteins.
We have recently discovered that one variant of PGC-1α is not present at all before exercise, but high levels of it can be found after only one hour of cycling.
This suggests that certain genes are turned on exclusively by exercise, and this may be a clue to the effects of exercise training on health. We are now investigating possible protein modulators of PGC-1α, which may attach to this protein to increase or decrease its activity in boosting mitochondrial protein production.
Epigenetic factors
We are also exploring the possible impact of exercise on epigenetics. Epigenetic changes affect how the DNA is used, without affecting the genetic information encoded within it. In our cells, DNA is wrapped around coin-shaped proteins called histones. Attaching small chemical molecules to the DNA strand or to histones affects the ability of transcription factors to reach their target genes. For example, adding a methyl (CH3) molecule to DNA generally makes the adjacent genes less accessible and thus less active, whereas attaching an acetyl (COCH3) group to histones usually relaxes that part of the DNA strand, making it more accessible for transcription (figure 5).
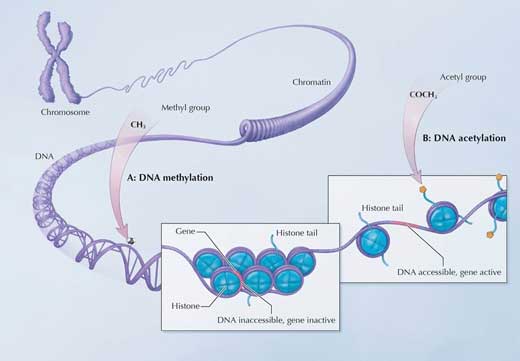
Image adapted with permission from the NIH Common Fund (for original, see: http://commonfund.nih.gov/Epigenomics/epigeneticmechanisms.aspx)
Using the biopsy material from our volunteers, we aim to see if such epigenetic effects remain after a prolonged period without physical training, and whether they influence how an individual responds to a later period of training. Based on the results of these experiments, we will be able to investigate whether ‘muscle memory’ truly exists and, if so, how this happens.
Acknowledgement
The authors would like to thank Associate Professor Carl Johan Sundberg for providing us with the opportunity to work in his lab and for valuable input on this article.
Resources
- To learn more about the role of exercise in the prevention and treatment of different diseases, see:
- Henriksson J, Sundberg CJ (2008) General effects of physical activity. In Ståhle A (ed) Physical Activity in the Prevention and Treatment of Disease pp 11-37. Stockholm, Sweden: Professional Associations for Physical Activity. ISBN: 9789172577152.
- WHO (2010) Global recommendations on physical activity for health. ISBN: 9789241599979.
- The publication is currently available in English, Chinese, French, Russian and Spanish.
- For more information about the physiological effects of exercise, see:
- Wilmore JH, Costill DL, Kenney WL (2007) Physiology of Sport and Exercise 4th edition. Champaign, IL, USA: Human Kinetics. ISBN: 9780736055833
- The BBC’s GCSE Bitesize website has a resource called ‘effects of training and exercise’. It is aimed at 14- to 16-year-old students.
- ‘How the body responds to exercise’ video on the US Teachers’ Domain website
- The following research articles provide more information about the scientific details:
- Booth FW, Gordon SE, Carlson CJ, Hamilton MT (2000) Waging war on modern chronic diseases: primary prevention through exercise biology. Journal of Applied Physiology 88(2): 774-787
- Gollnick PD et al. (1973) Effect of training on enzyme activity and fiber composition of human skeletal muscle. Journal of Applied Physiology 34(1): 107-111
- Norrbom J et al. (2011) Alternative splice variant PGC-1α-b is strongly induced by exercise in human skeletal muscle. American Journal of Physiology – Endocrinology and Metabolism 301(6): E1092-1098. doi: 10.1152/ajpendo.00119.2011
- Norrbom J et al. (2010) Training response of mitochondrial transcription factors in human skeletal muscle. Acta Physiologica198(1): 71-79. doi: 10.1111/j.1748-1716.2009.02030.x
Review
Exercising is an activity that relates (or should relate) to every human on the planet; as such, it has received a lot of attention for decades. Although the potential benefits of exercise are well known, the cause of these benefits is largely unknown. This article gives an overview of some of the genetic factors that might be involved in the positive outcomes observed in the physical state of an individual who exercises.
Biologists and other persons with at least a modest understanding of genetics, including advanced secondary-school biology students, will probably find the information provided in this article highly interesting. They might even be motivated to think of alternative ways in which exercising can lead to health benefits.
Michalis Hadjimarcou, Cyprus