The microbial fuel cell: electricity from yeast Teach article
We all know that yeast is used to produce beer and bread – but electricity? Dean Madden from the National Centre for Biotechnology Education, University of Reading, UK, shows how it works.
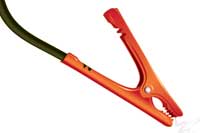
Image courtesy of Jobalou /
iStockphoto
Introduction
For decades, microbes that produce electricity were a biological curiosity. Now, however, researchers foresee a use for them in watches and cameras, as power sources and for bioreactors to generate electricity from organic waste. The microbial fuel cell described here generates an electrical current by diverting electrons from the electron transport chain of yeast. It uses a ‘mediator’ (in this case, methylene blue) to pick up the electrons and transfer them to an external circuit. This process is not very efficient, and this demonstration fuel cell will generate only a very small current. In the classroom, this can provide a stimulating introduction to the study of respiration and permit the study of some of the factors that influence microbial respiration. More recently, mediator-less fuel cells of greater efficiency have been developed, in which the micro-organisms donate electrons directly to the fuel-cell electrodes.
Equipment and materials
Needed by each student or working group
Equipment
- Perspex fuel cell, cut from 4 mm thick Perspex sheet
- 2 neoprene gaskets
- Cation exchange membrane, cut to fit between the chambers of the fuel cell. The membrane may be re-used indefinitely, but will melt if it is autoclaved.
- 2 x 10 ml plastic syringes, for dispensing liquids
- Petri dish base or lid, on which to stand the fuel cell
- 2 electrical leads with crocodile clips
- 0–5 V voltmeter or multimeter and/or low-current motor
- Scissors.
Materials
- 2 carbon-fibre tissue electrodes, cut to ?t inside the fuel cell
- 2 pieces of J-Cloth® or similar fabric, cut to ?t inside the fuel cell (the purpose of this cloth is simply to prevent the electrodes from touching the cation exchange membrane and short-circuiting the cell)
Important: All of the solutions listed below must be made up in 0.1 M phosphate buffer, pH 7.0, not in water.
- Dried yeast, made into a thick slurry in 0.1 M phosphate buffer (do not add glucose solution without first rehydrating the yeast in buffer)
- 5 ml methylene blue solution (10 mM)
- 5 ml glucose solution (1 M)
- 10 ml potassium hexacyanoferrate (III) solution (0.02 M) (also called potassium ferricyanide).
Procedure
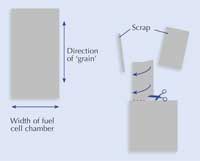
make the electrodes has a
‘grain’. To ensure that the
long ‘tail’ of the electrode
does not tear and that it ?ts
easily through the hole in
the fuel cell, it is necessary
to cut and fold the electrode
as shown here. Cut about
half-way through the upper
piece as shown, then fold
it in half, then half again to
form a ‘tail’ on the electrode.
Click to enlarge image
Image courtesy of Dean
Madden
- Cut out two carbon-fibre electrodes as shown in the image.
- Cut out two pieces of J-Cloth to ?t inside the fuel cell.
- Assemble the fuel cell as shown below.
Stand the assembled fuel cell on a Petri dish base or lid, to catch any liquid that may leak from the cell. - Combine equal (5 ml) volumes of the yeast slurry, glucose and methylene blue solutions. Syringe this mixture into one chamber of the fuel cell.
- Syringe potassium hexacyanoferrate (III) solution into the other chamber of the cell.
- Connect a voltmeter or multimeter (via the crocodile clips) to the electrode terminals. A current should be produced immediately – if the meter registers zero, check the connections and ensure that the carbon-fibre electrodes are not touching the cation exchange membrane.
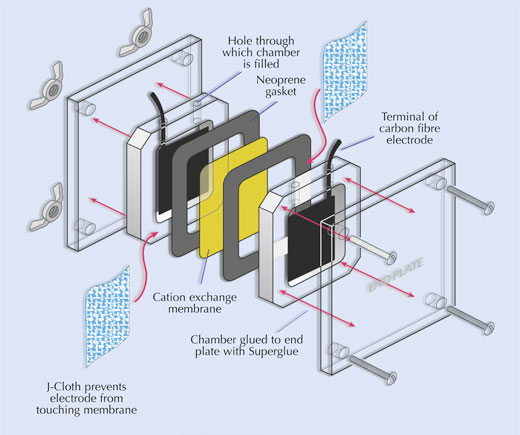
– the one shown here is roughly 55 mm x 55 mm)
Image courtesy of Dean Madden
Typical results
Microbial fuel cells of this type typically generate 0.4–0.6 V and 3–50 mA. If the cell is topped up with solutions as necessary, it will continue to generate electricity for several days.
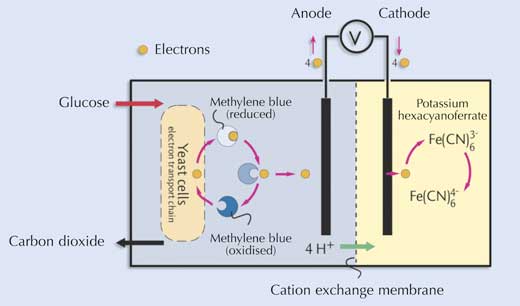
In one chamber of the cell, yeast cells are fed on glucose solution. A mediator,
methylene blue, enters the yeast cells and takes electrons from the yeast’s
electron transport chain. The electrons are then passed to an electrode (anode).
The electrons pass through the external circuit and are accepted by potassium
hexacyanoferrate (III) in the second chamber of the cell. Hydrogen ions pass
through a cation exchange membrane, which separates the two chambers.
Microbial fuel cells of this type typically generate 0.4-0.6 V and 3-50 mA.
This is sufficient to power a very low-current motor. If several such cells are
joined in series, it is possible to light a light-emitting diode (LED)
Image courtesy of Dean Madden
Safety
Potassium hexacyanoferrate (III) is poisonous. Eye protection should be worn when handling this material. If the solution comes into contact with the eyes, flood them with water and seek medical attention. If swallowed, give plenty of water to drink and seek medical attention. If spilled on the skin, the solution should be washed off promptly with water. Local regulations should be observed when disposing of used solution.
Recipes
To make 0.1 M phosphate buffer, pH 7.0, dissolve 4.08 g Na2HPO4 and 3.29 g
NaH2PO4 in 500 ml distilled water.
Preparation and timing
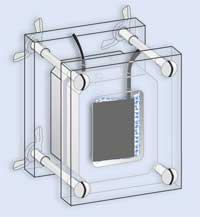
cell. Click to enlarge image
Image courtesy of Dean Madden
Solutions of the reagents may be prepared in advance. Note, however, that the glucose solution should be prepared no sooner than 24 hours before the work is to be carried out, as the solution is not sterile and would therefore support the growth of contaminating micro-organisms.
Pre-soak the cation exchange membrane in distilled water for 24 h before use.
Dried yeast can be rehydrated as the fuel cell is assembled, although it is important to add the dried yeast to buffer solution first, then to add glucose solution to the yeast slurry. If you try to rehydrate the yeast directly in glucose solution, osmotic effects will delay the process. (If using fresh yeast, simply make a thick slurry of that with the buffer solution before adding the glucose solution.)
It takes about 30 min from the assembly of the fuel cell to the generation of electricity.
Scope for open-ended investigations
Several fuel cells may be joined together in series to give a greater voltage; the current produced will remain the same, however. Conversely, increasing the size of the cell (or the electrode area) will increase the current generated, but not the voltage.
Different mediators and/or types of yeast, such as wine-makers’ or bakers’ yeast, may be used. Note that for safety reasons, the use of this fuel cell with other micro-organisms is not recommended.
Investigate the effect of temperature on the action of the fuel cell (remember to consider what ‘controls’ are necessary when making comparisons of this type).
Suppliers
Microbial fuel cells suitable for school investigations as described here are available from the National Centre for Biotechnology Education (NCBE) at the University of Reading, UKw1.
For those who prefer to build their own fuel cells, following the instructions in this article, the cation exchange membrane and carbon-fibre tissue electrodes are also available from the NCBE. The cation exchange membrane can also be purchased from VWRw2.
Low-current motors suitable for use with a fuel cell such as the one described here are expensive and difficult to find.
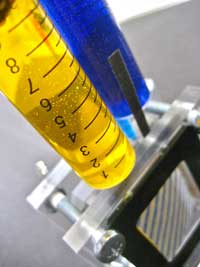
Image courtesy of Dean
Madden
Disposal of waste and recycling of materials
Potassium hexacyanoferrate (III) solution is poisonous. Local regulations should be observed when disposing of used solution.
Storage of materials
The potassium hexacyanoferrate (III) solution is light-sensitive and should therefore be stored in a light-proof bottle or in a bottle wrapped in aluminium foil. It should not be kept for more than six months.
You may wish to store the cation exchange membrane in a bottle of distilled water so that it is ready to use. The water should be replaced from time to time if the membrane is stored for an extended period.
Dried yeast, even in a sealed container, has a limited shelf life. The supplier’s ‘best before’ date should therefore be observed.
Acknowledgements
The microbial fuel cell was developed by Dr Peter Bennetto, formerly of the Department of Chemistry, King’s College, London, UK. It has been adapted for school use by John Schollar and Dean Madden.
Web References
- w1 – To learn more about the National Centre for Biotechnology Education (NCBE) and to order their fuel cells, see: www.ncbe.reading.ac.uk
- w2 – To contact VWR, the supplier of the cation exchange membrane, see: www.vwr.com
Resources
- Bennetto P (1987) Microbes come to power. New Scientist 114: 36–40
- Bennetto HP (1990) Electricity generation by micro-organisms. BIO/technology Education 1: 163–168. This article can be downloaded from the NCBE website: www.ncbe.reading.ac.uk or here: http://tinyurl.com/ncf6ql
- Lovley DR (2006) Bug juice: harvesting electricity with micro-organisms. Nature Reviews Microbiology 4: 497–508. doi: 10.1038/nrmicro1442
- Sell D (2001) Bioelectrochemical fuel cells. In: Biotechnology. Volume 10: Special Processes (Second edition). Rehm H-J and Reed G (Eds). Frankfurt am Main, Germany: Wiley-VCH. ISBN: 9783527620937
Review
This article describes a laboratory practical for demonstrating the electron transport chain. The practical is highly relevant for biology lessons on microbial respiration. It seems obvious to use this practical as an extension of fermentation exercises.
The practical can be used interdisciplinarily at the interface of biotechnology and physics, demonstrating the use of micro-organisms for energy production. It could also be related to the production of bioethanol, as an example of an alternative biotechnological way of producing energy.
Niels Bonderup Dohn, Denmark