Shipwreck: science to the rescue! Understand article
The Mary Rose is one of several famous and historical ships salvaged from the sea in recent decades. Thanks to the anaerobic conditions on the seabed, the remains are well preserved. Montserrat Capellas and Dominique Cornuéjols from the European Synchrotron Radiation Facility (ESRF), in Grenoble,…
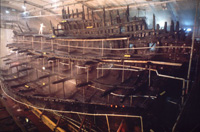
Rose
Photo courtesy of the Mary
Rose Trust
The Mary Rose
The Mary Rose, the flagship of the English Tudor king, Henry VIII, is the only 16th-century warship on display anywhere in the world. Built in Portsmouth and launched in 1511, she was the pride of the Tudor navy for 34 years until a battle with the French in 1545. The exact cause of the loss of the Mary Rose is unclear, although handling error appears to be most likely. Whatever the reason, as the ship leaned and the water entered the gun ports, her fate – and that of hundreds of men on board – was sealed. For over 400 years, she was buried in clay on the seabed at a depth of 14 metres off the south coast of England, until marine archaeologists salvaged her in 1982. The ship offers a rare insight into life at sea in Tudor times, but she needs to be carefully conserved.
The danger of sulphur and iron compounds
Sulphur compounds that have accumulated in the wooden structure of the ship pose the main threat to the salvaged Mary Rose. In seawater, bacteria feeding on organic matter, e.g. carbohydrates, (CH2O)n, reduce sulphate ions, SO42-, to hydrogen sulphide, H2S.
(CH2O)n + n/2SO42- → n/2H2S(aq) + nHCO3–
Dissolved hydrogen sulphide, H2S(aq), then penetrates the wood, where it is transformed into solid reduced sulphur compounds, such as thiols, R-SH (R denotes an organic part), in the lignin of the wood and to iron(II) sulphides when iron ions are available. Over the centuries, the reduced sulphur has accumulated; chemical analyses showed that the hull timbers of the Mary Rose now contain about 1% (in total, 2 tonnes) of the element sulphur (S).
Once the ship is removed from the water, the sulphur compounds in the wood slowly oxidise, forming sulphuric acid, H2SO4. The acid, if left untreated, could eventually degrade the cellulose fibres of the wood and thus decrease the stability of the hull timbers.
In the presence of iron ions, the threat of acid becomes particularly acute, because iron ions catalyse the oxidation processes and amplify the production of sulphuric acid. The wooden structure of the Mary Rose, like that of many other wrecked ships, contains iron from corroded iron bolts, nails and other objects on the ship. On the seabed, dissolved iron(II) ions have combined with the hydrogen sulphide to form iron sulphides, for example pyrite, FeS2, which is unstable when the moist wood is exposed to oxygen:
FeS2(s) + 7/2O2 + (n+1)H2O → FeSO4·n(H2O)(s) + H2SO4(aq)
The hydrated iron(II) sulphates melanterite, FeSO4·7(H2O)(s), and rozenite, FeSO4·4(H2O)(s), are commonly found as precipitates on sulphur-infested marine-archaeological wood.
X-rays to the rescue
X-rays are known for their medical use in imaging and radiotherapy, but can also help take care of vulnerable shipwrecks. X-rays can non-destructively analyse the elements in an object and their chemical state. To obtain such information for sulphur, the technique of choice is X-ray absorption spectroscopy. X-rays of a given energy are sent to the sample being studied, causing electrons in the inner shells of the sulphur atoms to be excited to higher energy or ejected as photoelectrons. Then either the kinetic energy of these photoelectrons is measured (in a technique known as photoelectron spectroscopy), or the X-rays emitted by the sample when the excited atoms return to their normal state (X-ray fluorescence) are analysed to measure the X-ray absorption.
In these ways, the binding energy of the excited electron can be obtained, which is characteristic of the type of sulphur compound. For the Mary Rose, it was essential to find the quantities and locations of the sulphur and iron compounds in the wood. Very intense X-rays, known as synchrotron light (see box), were needed to investigate the chemical composition of the Mary Rose in microscopic detail, and to deduce the best strategy to prevent her from further degradation.
Improving conservation
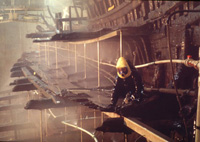
has been sprayed with a
solution of polyethylene
glycol for the past 10 years
to replace the water and
prevent cracking
Photo courtesy of the Mary
Rose Trust
For long-term preservation of the Mary Rose, it seems necessary to remove the strongly acid-forming iron sulphides from the wood. During the current conservation spray treatment, which began ten years ago, the acid is washed away as it forms (see photo). To more rapidly exhaust the acid-forming iron sulphides during spraying, mild oxidation by singlet oxygen (1O2, short-lived energy-rich oxygen formed by using ultraviolet radiation to excite a valence electron in an ordinary O2 molecule) could be tested. To increase the rate of the reactions, the temperature of the spray solution could be moderately increased, but the possible formation of Legionella bacteria then becomes a health hazard.
Recent investigations, including X-ray microscopic analyses at the European Synchrotron Radiation Facility, allowed researchers to suggest further steps. One method that is being tested removes iron by means of a complex-forming compound, or chelate, known as EDMA (ethylenediiminobis (2-hydroxy-4-methyl-phenyl) acetic acid). EDMA forms very strong bonds with iron(III) and dissolves the iron compounds. Even rust, FeOOH(s), forms water-soluble complexes in alkaline solution with EDMA and can be washed out of the wood. In the absence of iron, the remaining sulphur oxidises slowly and can be controlled with a stable climate and antioxidants.
Above all, it is important to monitor the effectiveness of the treatment, and X-ray analysis is an efficient way to evaluate the iron extraction procedure and the removal of specific sulphur compounds.
Synchrotron X-rays
In a synchrotron, electrons are accelerated until they reach a very high energy (six billion electron-volts at the European Synchrotron Radiation Facility (ESRF) in France). These high-energy electrons are then injected into a storage ring where they circulate in a vacuum at close to the speed of light. The synchrotron X-ray beams, emitted when the electrons change direction in strong magnetic fields, are directed towards the experimental stations that surround the storage ring.
The X-ray beams produced by the ESRF are a thousand billion times brighter than the beam produced by a hospital X-ray machine. As a result, these thin and very intense beams of synchrotron light can be used to resolve the structure of matter at a level of detail impossible to reach with standard X-rays. Synchrotron light sources can therefore be thought of as super-microscopes, and are used in numerous fields of research, including materials science, archaeology, biology and physics.
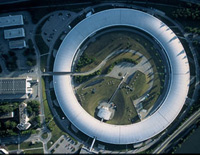
There are currently about 70 synchrotrons in the world. Various techniques, ranging from X-ray diffraction and small-angle scattering to photoelectron spectroscopy and X-ray absorption on a microscopic level, are available to an ever-growing number of scientists. These techniques are used to study matter in its different forms – as a crystal or a polymer, as biological or environmental samples, or as solid, liquid or gas.
Synchrotron X-rays are useful for analysing wrecked historical ships, where they are used to determine the quantities, location and chemical state of sulphur and iron compounds in microscopic detail. In this way, researchers can monitor the deterioration of the marine-archaeological wood and improve the methods of conservation.
For this purpose, Magnus Sandström and his team at the University of Stockholm, Sweden, have been travelling periodically to the Stanford Synchrotron Radiation Laboratory in California, USA, since 2001. They started by investigating why acid was forming in the timbers of the Vasa, a 17th-century Swedish warship, and have now extended their research to other historical shipwrecks, such as the Mary Rose. Last year, they gathered at the ESRF to carry out experiments to complement those performed at Stanford.
Extracting the iron compounds from shipwrecks
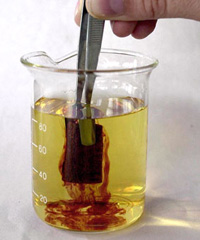
from the Vasa is submerged
in alkaline sodium-EDMA
solution, the iron compounds
in the wood dissolve in the
blood-red complex [FeIII-
EDMA]-.
Photo courtesy of Stefan
Evensen, The Vasa Museum,
Stockholm, Sweden. © Stefan
Evensen
Scientists investigating the Swedish warship Vasa, which sank on its maiden voyage on 10 August 1628, hit on the idea of dissolving the iron compounds present in the wood and removing them as water-soluble complexes. A chelate called EDMA is being tested. The sodium salt of the soluble iron complex, [Fe(III)-EDMA]-, is used in large quantities as an iron micronutrient in citrus agriculture, for plants that cannot extract iron from alkaline soil in which the iron is insoluble. The compound is used to fertilise orange groves in Spain and to grow lemons and grapes in Italy and France.
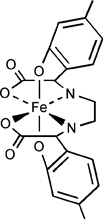
Comprehension questions
By Magnus Sandström
- The hull timbers of both the Vasa and the Mary Rose have been found to contain about 2 tonnes of sulphur, as the element S. If 1000 kg sulphur in the wood were in the form of the pyrite, FeS2, how much sulphuric acid (H2SO4(aq)) would be produced when all the pyrite is fully oxidised? Two pathways are common: to hydrated iron(II) sulphate:
FeS2(s) + 7/2O2 + (n+1)H2O → FeSO4·n(H2O)(s) + H2SO4(aq)
or to goethite, alpha-FeOOH (as in rust):
FeS2(s) + 15/4O2 + 5/2H2O → FeOOH(s) + 2H2SO4(aq)
- Sodium hydrogen carbonate (sodium bicarbonate, NaHCO3) has been added to the re-circulated sprayed conservation solution of the Mary Rose to keep its pH about 7. How much sodium hydrogen carbonate would be needed to neutralise the acid formed in Question 1 (from oxidised pyrite containing 1000 kg sulphur)?
- Crystalline pyrite has a volume of 40 Å3 per FeS2 unit and expands dramatically when oxidised. For example, the volume per formula unit of the crystalline hydrated iron(II) sulphate melanterite, FeSO4·7(H2O)(s), is 243.5 Å3 and of rozenite, FeSO4·4(H2O)(s), 162.7 Å3. Also natrojarosite, NaFe3(SO4)2(OH)6, with a volume of 266.0 Å3 per formula unit, is commonly found on the Vasa’s wood. Estimate how many times the volume will increase when a pyrite crystal oxidises and a) FeSO4·7(H2O)(s), b) FeSO4·4(H2O)(s) or c) NaFe3(SO4)2(OH)6 salts crystallise as products. What effects could these processes have if they took place inside the wood structure?
- In a chemistry textbook, look up a schematic molecular orbital energy level diagram for the oxygen molecule O2 in its ground state. Explain how uptake of energy from light can produce singlet oxygen, 1O2, with all electrons paired.
Answers are provided here.
Resources
- The Mary Rose can be visited in the Mary Rose Museum in Portsmouth, UK. Information about the ship, activity sheets, and details of visits are available on the Mary Rose Museum website.
- The Vasa is displayed in the Vasa Museum in Stockholm, Sweden. Details of the history of the Vasa, continuing research into its preservation, and how to visit are available on the Vasa Museum website.
- Details of the work of Professor Sandström and his group to preserve the Vasa and the Mary Rose are available here.
- The European Synchrotron Radiation Facility is an international facility which operates, maintains and develops the most powerful synchrotron light source in Europe with 18 participating countries. More than 5000 researchers come to the European Synchrotron Radiation Facility every year to use the light source and its associated instruments.
Institutions
Review
This article would be of great interest to teachers of the physical sciences and also to colleagues who teach history. It would be useful for teachers who wish to put more applications of chemistry and physics into their teaching and to promote more positive uses of the physical sciences in unusual situations. It would also serve as stimulus material for older students who could use the very helpful web links for further research into other scientific or historical aspects of this article.
I enjoyed the article. It stimulated me sufficiently to follow up some of the information given.
Tim Harrison, UK