Epigenetics Understand article
We tend to think of our genetic information as being encoded in DNA – in our genes. Brona McVittie from Epigenome NoE, UK, describes why this is only part of the story.
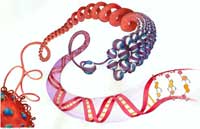
Image created by Nicolas
Bouvier; courtesy of Genevieve
Almouzni, Curie Institute,
Paris, France
How epigenetics shapes life
… it is tempting to wonder if this twisted sugar string of purine and pyrimidine base beads is, in fact, God.
James Watson
More than 50 years have passed since James Watson and Francis Crick first published the 3D structure of the DNA double helix. With Darwinian evolutionary theory now so widespread, the discovery that DNA encodes hereditary characteristics has proven popular. When Crick passed away in 2004, broad media coverage signified how much these concepts are accepted beyond the scientific community. However, we are coming to realise that gene-centric theories of evolution are limited in their scope. The genetic blueprint, like a complex musical score, remains lifeless without an orchestra of cells (players) and epigenotypes (instruments) to express it.
Science is now uncovering what plays our genetic score, and it appears that this performance can change dramatically between generations without any alteration to the DNA sequence. The field of epigenetics seeks to determine how genome function is affected by mechanisms that regulate the way genes are processed. Epigenetic factors include both spatial patterns, such as the arrangement of DNA around histone proteins (chromatin), and biochemical tagging.
There are hundreds of different kinds of cells in our bodies. Although each one derives from the same starting point, the features of a neuron are very different from those of a liver cell.
With some 30 000 genes in the human genome, the importance of silence, as in any orchestral performance, must not be underestimated. As cells develop, their fate is governed by the selective silencing of genes. This process is subject to epigenetic factors. Patterns of DNA methylation, the addition of a methyl group, play a role in all sorts of phenomena in which genes are switched on or off, from the splash of purple on a petunia petal to the growth of cancerous tumours.
Failure to silence genes can produce a hazardous cacophony. Abnormal DNA methylation patterns can alter the spatial arrangement of chromatin. This, in turn, affects which genes are silenced after cell division. Hypermethylation can inhibit the work done by protective tumour suppressor genes and DNA repair genes. Such epimutations have been observed in a wide range of cancers. These epigenetic insights are offering new therapeutic avenues for exploration.
Epigenetics also provides a means by which genetic material can respond to changing environmental conditions. Although plants do not have a nervous system or a brain, their cells have the ability to memorise seasonal changes. In some biennial species, this ability is linked to their capacity to flower in the spring, when warmer ambient temperatures are detected. Research has shown how exposure to cold during winter triggers structural changes in chromatin that silence the flowering genes in mouse-ear cress. These genes are reactivated in spring when longer days and warmth are more conducive to reproduction.
The environment can also prompt epigenetic changes that affect future generations. Recent laboratory studies on inbred mice demonstrated how changes to their diet might influence their offspring. Their fur can be brown, yellow or mottled depending on how the agouti gene is methylated during embryonic growth. When pregnant mothers were fed methyl-rich supplements such as folic acid and vitamin B12, their young developed mainly brown fur. Most of the babies born to the control mice (not given the supplements) had yellow fur.
Just as the conductor of an orchestra controls the dynamics of a symphonic performance, epigenetic factors govern the interpretation of DNA within each living cell. Understanding these factors could revolutionise evolutionary and developmental biology, and thus affect practices from medicine to agriculture. To answer Watson, “The genetic alphabet is more akin to the word of God, and its translation to His hand.”
Twin profiles
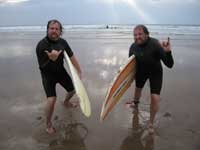
(left) and Gavin on the beach
“At times in their lives they have definitely striven to be perceived as a unit and at other times seem to want people to acknowledge their differences and respect them as individuals,” says Jim of his friends Gavin and Jason. Gavin and Jason are identical twins. One is literally a clone of the other.
Their similarities cannot be disputed, but when I first met them, I found them to be quite different people. Had I met them a few years earlier, I doubt I would have been able to tell them apart.
Monozygotic (identical) twins occur with an incidence of 1 in every 250 births worldwide. For reasons yet unknown, a fertilised egg cell clones itself and gives rise to separate embryos. Each will begin and end life with the same genetic make-up, but as they grow and develop they will experience differences in their environment, some of which might alter their appearance and behaviour.
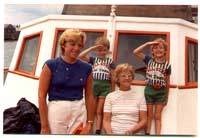
and Gavin with their mother
and grandmother
Gavin and Jason have exactly the same DNA. If one committed a crime and unwittingly left samples for forensic analysis, it would be impossible to determine the culprit from DNA fingerprint analysis. However, closer inspection of their molecules may reveal significant differences. Although the two share the same genes, recent evidence suggests that some genes might be active in one twin but not the other. They might be identical genetically but not epigenetically.
Such differences are discernable at the molecular level in the way that their chromosomes are arranged within the nucleus of each cell. Twisted around tiny protein (histone) balls, the same DNA string can have different consequences for a cell. Both balls and string assume complex 3D structures depending on their biochemical flavour. A variety of small molecules can affect the nuclear infrastructure by adhering to DNA and its associated histone proteins. Such flavours are influenced by the environment, most notably our diet.
Biochemical fine-tuning of the genome determines which genes are switched on, so twins are not necessarily destined to share the same fate. Recent research (Fraga et al., 2005) on around 80 different sets of monozygotic twins has revealed that their DNA is marked in different ways by a tiny molecule called methyl. So it’s not really true to say that they are identical. What’s more, these differences were much more pronounced in older than in younger twins.
This is new fodder for the age-old debate over how much the environment influences our fate relative to our genes. Although the similarities between identical twins are more striking than the differences, their inequalities could offer new avenues for disease research. For example, although Gavin and Jason are both at risk for type II diabetes, Jason was recently admitted to hospital with a pancreatic infection and had to inject himself with insulin for some time afterwards. The doctors were unable to make a more specific diagnosis. Had they been equipped with better diagnostic tools, such as those offered by new advances in epigenetic research, they might have been able to profile the twins to ascertain what was wrong with Jason.
References
- Fraga MF et al. (2005) Epigenetic differences arise during the lifetime of monozygotic twins. Proceedings of the National Academy of Sciences USA 102: 10604-10609. doi: 10.1073/pnas.0500398102
Web References
- Further information about epigenetics is available on the multilingual Epigenome NoE website
Review
Traditionally, identical twins are considered identical in all aspects of their make-up, right down to the way in which their DNA functions. The debate over nature versus nurture has persisted for many years, with many studies looking at identical twins who have been separated at birth and raised in different environments to see how they differ. At last there appears to be concrete evidence that nurture or environmental pressures influence how DNA is methylated or silenced in the genome, leading to minute changes in gene expression. These epigenetic changes, affecting the arrangement of DNA around histone proteins and its biochemical tagging, can be influenced by something as simple as diet or, in plants, by seasonal changes. The discovery of this phenomenon means that identical twins could, for example, react differently to the same medicine. Investigating how genome function is affected by gene silencing is thus an emerging and important field.
Although the control of gene expression in terms of the ‘lac’ operon is included in some syllabuses, DNA methylation as a mode of gene control is not considered within the UK A-level biology syllabus. It is mentioned in text-books in the context of bacterial DNA being methylated to prevent its own restriction endonucleases from cutting endogenous DNA. The idea of silencing genes by methylation could be introduced into genetics lessons as ‘how identical are identical twins?’ Most students should be aware of the nature versus nurture debate in the upbringing of twins, but this can now be extended. It may also be possible to introduce the concept of DNA methylation inactivating one of the X chromosome in females.
Shelley Goodman, UK