Is there anybody out there? An ark of life Teach article
An enormous meteorite impact and then a rocky flight from Mars. Is that how life appeared on Earth? Cornelia Meyer takes us on a space trip through the lithopanspermia theory and describes how she is putting it to the test with the help of student colleagues.
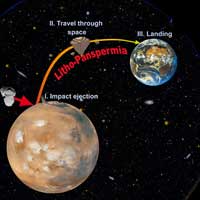
Mars image courtesy of NASA;
Earth image courtesy of the Deutsches
Zentrum für Luft- und Raumfahrt
On 7 August 1996, scientists at NASA announced they had identified structures that looked like microscopic fossil bacteria in the Martian meteorite ALH84001, found in Allan Hills, Antarctica. Although scientists disagree about the significance of the Allan Hills meteorite, the question remains: was there life on Mars?
When comets and asteroids strike planets, they can dislodge rock fragments that are catapulted into space and – like the Allan Hills meteorite – sometimes land on other planets as meteorites (see glossary). This has caused much speculation. Could the first life forms have arisen not on Earth but on Mars, or perhaps another distant planet? If so, could meteorites then have transported life to Earth?
In 2007, three other postgraduate students – Ralf Moeller, Thomas Berger and Jean-Pierre de Vera – and I decided to investigate this idea, known as the lithopanspermia theory (see image above), in three steps:
- The ejection of living organisms into space, on board a meteorite.
- The effect of space travel on living organisms.
- Their survival as they enter Earth’s atmosphere and land.
The lithopanspermia theory – solid as a rock?
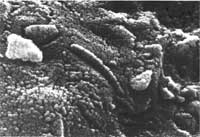
microscope image showing an
unusual tube-like structural form
that is less than 1/100th the width
of a human hair in size, possibly
representing the remains of
extraterrestrial bacteria, found in
meteorite ALH84001, a meteorite
believed to be of Martian origin
Image courtesy of NASA
The lithopanspermia theory (from the Greek: lithos = rock, pan = all, sperma = seed) was proposed in 1903 by the Swedish scientist Svante Arrhenius. Although the idea is not widely accepted, there is some evidence to support it:
- The existence of lunar and Martian meteorites on Earth
- The presence of organic material and (possibly) microbial fossils on the Allan Hills meteorite (see image)
- The fact that large comets or asteroids hitting a planet launch pieces of rock with enough velocity to overcome gravity and leave the planet (as meteoroids)
- The ability of bacterial spores to survive the shock waves caused by such an impact
- The UV-resistance of micro-organisms at the low temperatures found in space
- The survival for millions of years, in amber or salt, of bacterial spores
- The survival of bacterial spores in space for up to six years
- The palaeogeochemical evidence for ancient microbial ecosystems on Earth, leaving only about 400 million years for the evolution from simple precursor molecules to cellular life.
Glossary
Asteroid: One of the numerous small rocky bodies in orbit around the Sun. Most asteroids reside in the ‘main belt’ between Mars and Jupiter, but some have orbits that cross Earth’s orbit and could strike its surface.
Comet: One of the primitive icy bodies originating in the outer reaches of the Solar System that are in elliptical orbits around the Sun. Near the Sun, icy material vaporises and streams off the comet, forming a glowing tail.
Meteorite: An extraterrestrial rock that has fallen to Earth. Most meteorites are pieces of asteroids and are made of stone, stone and iron, or iron.
Meteoroid: A small solid body moving through interplanetary space. After falling to Earth it is called a meteorite.
1. The journey begins
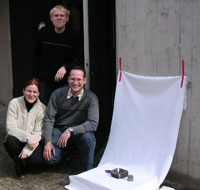
and Jean-Pierre de Vera
Image courtesy of Cornelia Meyer
As part of our Masters and PhD theses, we investigated the feasibility of the first step: the ejection phase, in which living material is launched into space by a meteorite impact (Horneck et al, 2008; Stöffler et al, 2007). To simulate the event, we took two slices of rock thought to be similar to the rocks on Mars, put a layer of micro-organisms between them, placed this ‘sandwich’ in an iron cylinder and blasted it with TNT.
We had good reasons for using micro-organisms in this experiment. On Earth, only microbes are known to survive in extremely hostile environments, so they were more likely to endure the experiment. Also, as simple organisms, they may be similar to the putative first life forms on Mars. The particular micro-organisms chosen for the experiment were bacterial spores, cyanobacteria and lichens that live inside or on rocks, and are known to survive simulated space conditions.
We also chose the rocks carefully. To discover whether a meteorite originates from Mars, its composition is compared with that of rocks studied on the surface of Mars. The most frequent Martian meteorites found on Earth are known as basaltic Shergottites, and are formed by volcanic activity. For our experiment, therefore, we used basalt: readily available on Earth and similar to Martian rock.
In repeated experiments, the TNT explosions exposed the micro-organisms to pressures of between 50 000 and 500 000 bar. These are similar to the pressures that would be generated by meteorite impacts on Mars, causing craters of more than 75 km in diameter and launching Martian rocks into space. The compression of the blast also exposed the micro-organisms to temperatures of up to 1000 °C. Although such conditions might be expected to destroy all life, at 400 000 bar (400 000 times normal air pressure), 0.02% of the micro-organisms survived.
Today, temperatures on Mars range from -143 °C at the poles to +27 °C at the equator. Although early Mars would have been warmer than it is today, it would have cooled down faster than Earth because it lost its atmosphere. This means that by the time of the proposed transfer of life from Mars to Earth (up to 20 million years ago), Mars would already have reached the low temperatures that exist there today. Therefore, in a second experiment to better reflect conditions on Mars, we used dry ice (solid carbon dioxide) to cool the apparatus to -80°C before blasting it, and found that some of the micro-organisms survived even at 500 000 bar. In the previous, uncooled, experiment, none had survived at that pressure.
During the experiments, the micro-organisms were exposed to the high temperatures and pressures only for a few microseconds, as would happen with a real meteorite impact on Mars. This may have been the key to their survival. So, the first part of the lithopanspermia theory appears to be plausible: organisms on rocks could survive the launch into space.
2. Space travel: the ESA SUCCESS student contest
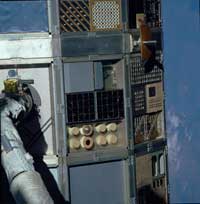
on top of the ISS, similar to the
artificial meteorite planned
Image courtesy of Cornelia Meyer
Next, we decided to compete for the opportunity to investigate step two of the lithopanspermia theory: could living organisms survive the extreme cold, cosmic radiation and vacuum during a long space journey? In the SUCCESS student contestw1, organised by the European Space Agency (ESA), we were offered the opportunity to fly an experiment on board the International Space Station (ISS) in November 2009.
Since the 1980s, several experiments have shown that micro-organisms are capable of surviving in space (e.g. Mileikowsky et al, 2000). However, the micro-organisms in those tests either were shielded from radiation by aluminium, or only spent a few days in space. So how long could they survive in space? We want to use the ISS for a more realistic investigation into the effect of space conditions on living organisms.
We suggested building an artificial meteorite packed with micro-organisms as well as sensors to measure cosmic rays and temperature. A piece of basaltic rock will be cut into eight slices, with holes for the micro-organisms and sensors. The holes will be sealed with rock, and the slices fitted back together into an airtight structure. The artificial meteorite will then be transported to the ISS, mounted onto an aluminium platform outside the Station and exposed to space conditions for six months. As a control, a second artificial meteorite will remain on Earth.
Once the meteorite returns to Earth, the biologists Ralf and Jean-Pierre will determine the survival rate of the micro-organisms and look for physiological changes induced by the conditions in space. As the mineralogist on the team, I will investigate the effects of space weathering on the artificial meteorite. Space weathering is a blanket term for processes, including cosmic radiation, solar winds and meteorite bombardment, that act on bodies in the harsh space environment. We will also compare the physical properties of the artificial meteorite with those of the rock that remained on Earth.
Besides providing evidence that may support the lithopanspermia theory, these results could supply information about the effect of space weathering on the optical properties of rock. These properties are important for the observation of asteroids, as optical spectroscopy is used to determine their elemental composition. Knowing more about the effects of space weathering, therefore, could help scientists to determine whether meteorites found on Earth and asteroids observed in space come from the same parent bodies.
3. A new experiment? The soft landing
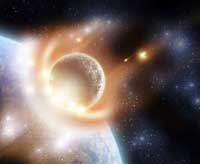
Image courtesy of iStockphoto
Even if first two parts of the lithopanspermia theory are plausible – micro-organisms could survive take-off from their home planet and a lengthy journey through space – could they survive on another planet? Astrobiologists at the Deutsches Zentrum für Luft- und Raumfahrt have suggested that Earth micro-organisms could survive for some time on Marsw2. This suggests that Martian life forms might also be able to survive on Earth, assuming they could withstand the impact. So far, however, we know very little about what would happen if a meteorite carrying living organisms landed on Earth. We do, however, have information that enables us to speculate.
When objects enter Earth’s atmosphere at high speed, their surfaces are exposed to very high temperatures due to friction. However, although temperatures in the outer layers of the meteorite are high enough to melt – or even vaporise – the rock, the inside of the meteorite remains closer to the -273 °C (0 K) found in space.
Very often, meteorites break up when they hit the ground. If any organisms survived inside the meteorite – protected from the very high temperatures at the surface – they would thus be released and could begin to colonise Earth. They would experience a temperature shock, coming from -273 °C in the meteorite core to the ambient Earth temperature, but micro-organisms are known to be able to survive rapid changes in temperature.
The evidence for lithopanspermia?
Although micro-organisms could have survived all three steps described in the lithopanspermia theory, this is not evidence that life on Earth has an extraterrestrial origin. Above all, we don’t actually know whether life exists beyond our planet – but the search for extraterrestrial life continues. And speculation about our origins continues, too.
The ESA SUCCESS contest
SUCCESS, the Space station Utilisation Contest Calls for European Student initiativeS, organised by ESAw1, aims to make today’s students into tomorrow’s users of the International Space Station (ISS). European university students up to Master’s level or equivalent, from any discipline, are invited to propose an experiment to fly on board the ISS.
The first prize is a one-year internship at ESA’s space research and technology centre ESTEC in the Netherlands. The winner will be able to work on their experiment, to enable it to fly to the ISS.
The contest is currently closed for new contestants. A new SUCCESS Student Contest is foreseen for 2010.
References
- Horneck G et al (2008) Microbial rock inhabitants survive impact and ejection from host planet: first phase of lithopanspermia experimentally tested. Astrobiology 8: 17-44
- Mileikowsky C et al (2000) Natural transfer of viable microbes in space. Part 1: From Mars to Earth and Earth to Mars. Icarus 145: 391-427
- Stöffler D et al (2007) Experimental evidence for the potential impact ejection of viable micro-organisms from Mars and Mars-like planets. Icarus 186: 585-588
Web References
- w1 – Details on the ESA SUCCESS student competition can be found here: www.esa.int/esaHS/SEMU9TGHZTD_education_0.html
- w2 – For more information about the Deutsches Zentrum für Luft- und Raumfahrt, see: www.dlr.de/en
- For details of their work on whether micro-organisms can survive on Mars, see: www.dlr.de/me/en/desktopdefault.aspx/tabid-2016
- w3 – For more information about the Museum of Natural History in Berlin, Germany, see: www.naturkundemuseum-berlin.de/index_english.html
Resources
- Astrobiology Lecture Course Network: http://streamiss.spaceflight.esa.int/?pg=production&PID=alcn
For more information about panspermia, see:
- www.karlsims.com/panspermia.html
- www.archive.org/details/sims_panspermia_1990
- Hartevelt S, Walker C (2008) The International Space Station: a foothold in space. Science in School 9: 62-65.
- Marinova M (2008) Life on Mars: terraforming the Red Planet. Science in School 8: 21-24.
- Wegener A-L (2008) Laboratory in space: interview with Bernardo Patti. Science in School 8: 8-12.
- Warmbein B (2007) Down to Earth: interview with Thomas Reiter. Science in School 5: 19-23.
- Warmbein B (2006) Launching a dream: the first European student satellite in orbit. Science in School 1: 49-51.