Fighting malaria on a new front Inspire article
Stéphanie Blandin explains her work on malaria to Russ Hodge from the European Molecular Biology Laboratory in Heidelberg, Germany – and describes how she became a molecular biologist.
The closest that many of us will ever come to malaria is a handful of pills prescribed before a trip to a tropical country, or the stories of ancient expeditions wiped out by mysterious fevers. But the disease seems remote only from the comfortable armchairs of the developed world. For much of the rest of the globe, malaria is still a huge problem. It kills millions each year – mostly children in Africa.
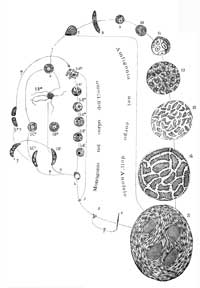
parasite (Giovanni Grassi,
1854-1925)
Malaria is caused by a single-celled parasite called Plasmodium. Scientists believe that the most dangerous form of this organism has been around for about eight million years – ever since humans diverged from apes. The disease probably became a huge problem when humans began clearing land for agriculture, leaving standing pools of water that made ideal breeding grounds for mosquitoes, which transmit the parasite to humans through a bite.
In our bodies, Plasmodium infects blood cells and reproduces, bursting out in waves. The surface proteins of the parasite change with each generation, helping it escape our immune system. An infection leads to fever and often death.
There are two main ways to fight malaria: first, by attacking mosquitoes – destroying the places where they breed or destroying the insects themselves with pesticides (such as the infamous, and now largely banned, chemical DDT). The second approach is to treat infected patients with drugs such as quinine, but Plasmodium is developing resistance to drugs. And global warming may bring malaria back to cooler regions where it has been almost entirely eliminated.
So researchers like Stéphanie Blandin, at the European Molecular Biology Laboratory (EMBL) in Heidelberg, Germany, have been putting new kinds of science to work on the problem. Studies of Plasmodium may reveal weaknesses in the organism that can be targeted with drugs. Another approach, in which Stéphanie is most interested, might be to take the fight inside the mosquito. Malaria makes the insects ill, too, and curing them would be just as good as stopping the organism in the human body – maybe even better.
Stéphanie has always wanted to be a scientist. Her father is a farmer who raises cattle and grows grain in the Bourgogne region of France; her uncles produce wine; her mother is a nurse. “At home, there were magazines lying around; I particularly remember a magazine about cancer research,” she says. “When I was very small, I liked to look at the pictures. I liked the bottles, the beakers and the laboratory equipment. I liked the strange bluish and purple colours – you know, the colours of the gels that we use in experiments. Then when I learned to read, I liked the strange names of the drugs they talked about.”
In school, Stéphanie liked mathematics and says she probably would have become a mathematician if she weren’t a biologist. She enjoyed the farm and the outdoors and considered going into agricultural science. Both plant and animal science were covered in the prépa – a school that some French students attend after high school, to prepare them for the grandes écoles where they will learn science and other careers.
Finally, she decided to concentrate on biology. “But first I wanted to see what real-life work in a lab was like. I also wanted to learn better English.” Stéphanie spent a few weeks in a laboratory at EMBL. Then when an opportunity opened up to do a practical period in the lab of Max Cooper at the University of Alabama, she jumped at the chance to go to the USA. Cooper was working on a fascinating problem: how molecular signals trigger generic cells to become specialised immune system cells. After six months in Alabama, she was hooked.
Stéphanie seems entirely at home in front of the microscope, mounting a slide and adjusting the focus with a quick twist of her hand. She steps back and lets me take a look. A few days ago, a mouse was bitten by Anopheles mosquitoes; we are looking at samples of its blood. The view is full of pearly globes – red blood cells – and when Stéphanie tells me what to look for, even I can see that the mouse has malaria. Some of the cells are cluttered with black spots, caused by a dye that marks the presence of the parasite.
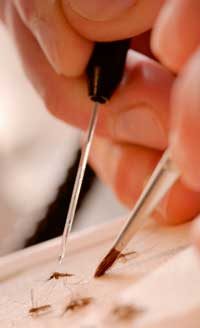
The hardest part of her job is to infect ‘cute white mice’ with malaria, she says. “But it’s necessary. If you have ever seen a child suffering from this horrible disease… that sight stays with you. The fact that the parasite is developing resistance to the drugs puts millions more people at risk and we are going to need new ways to fight it. It makes you work harder and helps you to make some of these difficult choices.”
Now that she has shown that the mice have been infected, Stéphanie will try to infect a new round of mosquitoes. She divides the insects into three hand-made cups that she designed herself – “mosquito houses” – because there are slight differences between the three groups. She will let them all feed on the infected mice – which have been briefly put to sleep – hoping to see differences in their ability to transmit the disease.
“When I began working in this area, almost nothing was known about the mosquito immune system – it wasn’t even certain that they really had one,” Stéphanie says. “There were good reasons to think that they might, because some other insects are known to have a basic immune system, and mosquitoes are related to them through evolution. Some flies, for example, produce proteins that protect them from some types of bacteria. Elena Levashina, my supervisor in the lab, had found two types of molecule called thioester-containing proteins [TEPs] in the mosquito. My job was to try to find more, and to discover whether they had anything to do with a mosquito immune system.”
Some mosquitoes seemed to be able to battle malaria: researchers had discovered a strain of Anopheles that was unable to transmit the disease. “This unusual strain is so close to the normal, disease-carrying type of mosquito that we think only a few mutations in genes – maybe even only one – protects it,” Stéphanie says. “A single protein might be killing the parasite in the mosquito body.”
Within two years, Stéphanie and her colleagues had found over a dozen other potential immune-system molecules. Early studies showed that TEPs helped mosquito cells kill bacteria – could the molecules also help in the fight against malaria? “You can’t study parasites and mosquito cells in the test tube,” Stéphanie says, “so we had to go directly to studies in the mosquito body.” The team discussed how to do these experiments, but they knew they were running up against the limits of gene technology.
“In other organisms, there are methods that let you shut down molecules or switch them on,” Stéphanie says. “This kind of genetic engineering is very useful to investigate such questions – you could take the immune strain and then shut down one of its proteins. If the mosquito could then transmit malaria although it hadn’t been able to before, we’d know that this particular molecule was helping to protect it.”
Unfortunately, these methods hadn’t yet been developed to work in the mosquito. A new method was being used in worms and other organisms to shut down specific proteins; it involved injecting animals with a slightly altered version of one of their own molecules. To create proteins, cells make a template molecule called RNA. Scientists had discovered that injecting cells with a modified version of the template for a specific protein would block the production of that protein. So Stéphanie tried the same method (called RNA interference, or RNAi) in the mosquito, and discovered that it worked very well. Then she could inject mosquitoes with modified RNA, shutting down specific TEPs, and hoping to knock out the immunity of mosquitoes resistant to malaria.
“I did exactly what I am showing you now,” she says. In her first ‘mosquito house’, she has controls – normal insects that should transmit malaria. The second and third houses hold mosquitoes that are normally resistant. But she has injected them with RNA one-by-one under the microscope, blocking crucial immunity proteins. Each of her two experimental strains has had a different TEP shut down. She hopes that at least one of the two altered types of mosquitoes will now be able to transmit the disease.
When the feeding is done, she returns the sleeping mice to their cage and the well-fed mosquitoes to their shelf. “Now the parasite has to grow and develop in the mosquito,” she says. “We have to wait several days. The first time we did this, I was pretty excited.”
The one thing Stéphanie likes most about science is the freedom. You can establish your own rhythm and work the hours that you choose – normally a lot. She likes to come by in the evening: quiet hours, when most people have gone home – that’s when she gets the most work done. Often she comes in at noon and stays in the lab until two or three a.m. “It makes things a bit hard when your partner is also a scientist,” Stéphanie says; her husband also works at EMBL. “You have to try to find a common schedule.”
A few days after my first visit, she goes into the cold room next to the lab of Fotis C. Kafatos, where she has worked for the past few years. The mosquitoes she infected a few days ago are on ice. Below a certain temperature, the insects enter a dormant state in which they can survive a few days. She will now dissect them and search for traces of Plasmodium.
With a few deft movements under the microscope, she removes the mosquito’s mid-gut, a stringy white tissue that looks like a very tiny version of our own intestines. The meal of blood passes through the mid-gut as the mosquito digests its food, and it’s also here that the parasite starts its invasion. Plasmodium parasites in the blood squeeze through the cells that line the gut and travel through the mosquito body to reach the salivary glands, where they can infect a new animal or a human when the mosquito next feeds.
Her test has been successful. Stéphanie finds traces of the live parasite in strains of mosquitoes that normally can defeat Plasmodium. By shutting down a single type of TEP, she has succeeded in transforming non-infectious Anopheles into carriers of malaria.
“Obviously what we want to do is the opposite,” she says. “Now that we know this particular protein, TEP1, is vital to killing the parasite, we can look for ways to introduce it into mosquitoes that otherwise transmit the disease.” It will probably be many years, she says, before this work will result in a cure for malaria. That might take the form of a ’smart spray‘ that strengthens the mosquito immune system, or even result in genetically modified mosquitoes that might one day be released into the environment. Before any of that happens, of course, scientists will have to know a lot more about Anopheles mosquitoes, and will only intervene after careful studies and with the full consent of affected countries.
No one knows where the next major breakthrough in the fight against malaria will come from. But in the past, the key battles against the disease have been won by taking the fight to the mosquitoes. As long as the disease exists, and they are able, insects will continue to transmit malaria. That’s more than enough reason, Stéphanie and her colleagues think, to attack the problem with all the tools available to today’s molecular biologist.
Institutions
Review
This article talks about a scientist who works in a molecular biology lab, trying to find a cure for the deadly disease of malaria. The end result is a story any scientist – or anyone thinking about becoming a scientist – should read. It is interesting, optimistic and sends a message of hope for a better world. At the same time, it raises many important questions.
As the story unfolds, the reader gets a general overview of the specifics of the disease: how it is caused, how it is spread, what efforts have been used to battle it so far, and what the present research focuses on. Although much of this information can be found in many good biology schoolbooks, it is presented here in an interesting and readable fashion.
When you read about the scientist in the article, you get a positive view of what scientists do: they have the opportunity to work in research centres around the world carrying out fascinating projects; they use modern scientific techniques; they are free to design their own (admittedly quite heavy) work schedule; and most importantly, their personal contribution can lead to discoveries of worldwide significance, which could save the lives of millions, especially children.
Perhaps what makes this article unique is that it effortlessly raises a number of topical issues, which concern not only the scientific society but also governments, organisations and the general public. In a very short historical account of the appearance of malaria, the author hints that perhaps it was human action thousands of years ago that allowed the disease to evolve from a rare one to a major killer. In modern times, human action resulting in global warming might even bring the disease from distant tropical countries right to the doorstep of the developed world. The issue of the use of lab animals in research is also briefly addressed. In addition, it is suggested that scientific discoveries should only be exploited after careful examination of all their implications.
This article could be used in various ways as a teaching aid. Apart from its obvious use as a source of scientific information for the teacher, it could be used as an alternative form of teaching tool. For example, the teacher could give it to the students and ask them to complete a worksheet to collect scientific information and obtain an insight into how real-life scientists design their research.
Michalis Hadjimarcou, Cyprus